The Quest for Mars: NASA scientists and Their Search for Life Beyond Earth
Laurence Bergreen
This edition does not include illustrations.Is there life on Mars? And if not, why not? These questions have gripped mankind throughout the twentieth century. In the shadow of the new millennium, The Quest for Mars seeks the definitive answers from the dedicated NASA scientists participating in the race to discover life on the Red Planet.‘Ever since I was a small child, I’ve believed there was life out there. When I look at the magnitude of the universe, with its billions of stars, I believe that if life developed here on Earth, it must have developed elsewhere. We simply can’t be unique. I really don’t think we’re the most intelligent life forms in the universe, but that’s just my gut feeling’, Dr Claire Parkinson, NASA scientist.Of all the planets, Mars has exerted the most powerful allure over the human intellect and imagination. Generations of astronomers have expected to find clues to the origin and destiny of Earth and its inhabitants concealed amid the red storms sweeping across the surface of Mars. Today, the mystique of Mars is even greater. Public interest in the Mars mission is sky-high; the exploits of the tiny Mars Rover ‘Sojourner’ in the summer of 1997 excited the greatest curiosity in a space mission in a generation.In The Quest for Mars Laurence Bergreen has unrestricted access to a team of NASA employees – engineers, geologists and other scientists – who are consumed by the search for proof of life on Mars. As one formidable obstacle after another attempts to scupper their quest for a deeper understanding of life on Mars and throughout the Solar System, the narrative takes us step by step through the exhilaration and the despondency of their extraordinary adventure. Nothing is off limits in this unique, behind-the-scenes story of space exploration.
The Quest for Mars
The NASA Scientists and Their
Search for Life Beyond Earth
LAURENCE BERGREEN
DEDICATION (#ulink_d649ee97-438a-507d-b4a6-1e5c5a4c0126)
To Betsy, Nick and Sara
EPIGRAPH (#ulink_b472da94-a837-5014-a9ff-cbff78c24261)
“Ever since I was a small child, I’ve believed there was life out there. When I look at the magnitude of the universe, with its billions of stars, I believe that if life developed here on Earth, it must have developed elsewhere. We simply can’t be unique. I really don’t think we’re the most intelligent life form in the universe, but that’s just my gut feeling.”
Dr. Claire Parkinson, NASA Scientist
CONTENTS
Cover (#uedaca595-9b3b-5619-8731-56fe35e82fdf)
Title Page (#u2b989433-b922-55d3-9885-a7a5c96cc684)
Dedication (#u63d9775a-b39b-5902-a268-71fdcdc82bc0)
Epigraph (#uf07b8d79-de56-5994-8898-606252215077)
PART ONE: THE MISSION (#u421d7e84-9e29-5b89-9b9d-37bf30b1c2c1)
1. Mars on Earth (#u25fcc64f-6889-57aa-9b5c-14e716d45905)
2. Message in a Bottle (#uea469cc6-4a2a-5425-a69f-bb8fa72e3161)
3. Ground Truth (#ufbde391e-6f96-5229-9952-0dea84cd7a84)
4. From Outer Space to Cyber Space (#ud9cff58a-8b46-5297-8fae-df68075d5e4e)
PART TWO: CODE S (#litres_trial_promo)
5. Shoot Out at Cal Tech (#litres_trial_promo)
6. The Honor of the Team (#litres_trial_promo)
7. Goddard (#litres_trial_promo)
8. The Genesis Question (#litres_trial_promo)
PART THREE: DISCOVERING MARS (#litres_trial_promo)
9. Rocket Science (#litres_trial_promo)
10. Ghosts and Ghouls (#litres_trial_promo)
11. Human Error (#litres_trial_promo)
12. Mars or Bust (#litres_trial_promo)
Epilogue: Stargazing (#litres_trial_promo)
Keep Reading (#litres_trial_promo)
Select Bibliography (#litres_trial_promo)
Index (#litres_trial_promo)
Acknowledgments (#litres_trial_promo)
About the Author (#litres_trial_promo)
Praise (#litres_trial_promo)
Also by the Author (#litres_trial_promo)
Copyright (#litres_trial_promo)
About the Publisher (#litres_trial_promo)
PART ONE (#ulink_dd9051c6-63b8-5ae5-bd64-2bce5b5edecd)
1 MARS ON EARTH (#ulink_7800e4c0-6a5d-5849-853a-0cefaa0598fc)
Subject: ICELAND
Date: Thu, 16 Jul 1998 00:48
From: Laurence Bergreen
To: Jim Garvin
Hi Jim,
It’s late Wednesday night, and I am back home from Houston. With time growing short, what can you tell me about Iceland? Last I heard, there was a strong chance of postponement till October. Looking forward to hearing from you as soon as possible. Thanks.
Larry
Subject: Re: ICELAND
Date: Thu, 16 Jul 1998 09:25:53
From: Jim Garvin
To: Laurence Bergreen
Larry,
We’re GO for Iceland. As of now, we are booked to arrive in Iceland early on the 20th, and quickly pick up a helicopter ride to Surtsey for a 6-hour working visit.
I am trying to be sure we can catch the Iceland Coast Guard helicopter, as we land between 6 and 6:30 AM and must get thru customs and get the rental Jeep.
Get set for Mars on Earth.
Jim
It’s 6:15 in the morning when Jim Garvin, a planetary geologist who works for the National Aeronautics and Space Administration, meets me at Iceland’s Keflavik Airport. As arranged, he’s flown in from Baltimore, and I’ve come from New York. Jim is forty-one, talks in torrents, and is plainly Type A, endowed with the passion and restlessness of an old-fashioned genius. Although he has two small children, he puts in eighty-hour work weeks. He is intense. There is no such thing as a short conversation with Garvin. His replies to simple questions have a way of digressing into hour-long ruminations on the nature and origins of the universe, but he gets away with it mostly because he is unfailingly polite. Once he launches into a monologue, he gestures emphatically, as if visualizing and touching everything he describes. He is fit and compact, with black hair, handsome Irish features, and a perpetually worried voice. He looks clean-cut, at least compared to other scientists, and his skin is slightly irritated in patches, as though he’s been vigorously applying after-shave lotion. A friend once told me it is often hard to get Jim Garvin’s attention, but once you do, it can be overwhelming. Now I have his attention.
After we retrieve our bags, Jim sets out to find Oscar, the pilot of the plane we’ve hired to take us from Keflavik to the island of Heimaey, off the southern coast of Iceland, where we are to rendezvous with the Iceland Coast Guard, weather permitting. Oscar, when we catch up with him, looks too young to drive a car, let alone pilot a plane. We cram ourselves into his single-engine Aerospatiale, a lightweight aircraft of French design. The co-pilot’s seat I occupy is so cramped that my knees interfere with the controls. We are battling fatigue, Jim and I. We have been up all night, and the inside of my mouth tastes like kerosene from the Aerospatiale’s tank.
We have come all this way because geologists studying Mars have designated Iceland a Mars analogue. In 1976, when the Viking Lander returned color images of the Red Planet, scientists realized that Mars bears a striking resemblance to the landscape sliding below Oscar’s little airplane. Iceland is, in many places, an arctic desert devoid of vegetation and untouched by humanity. These days, NASA-supported scientists regularly visit to study this volcano-ridden island to compare it to its distant relative, Mars. The theory is that by studying Iceland, scientists can better understand the workings of the Red Planet. Iceland is only twenty million years old, a geological babe, and thus relatively unweathered, a primeval landscape. The absence of trees on the Icelandic landscape is a blessing, revealing the island’s geological makeup. Mars is similarly bare. Iceland festers with active and dormant volcanoes – just as Mars does. The resemblance makes it possible to work out significant aspects of the geologic history of both places by comparing the two.
Mars is so reminiscent of Earth that it is considered “semi-habitable.” The atmosphere is only one percent as dense as ours, but breathable air could be extracted from it. The Martian day, or “sol,” lasts about as long as a day on Earth; a Martian year consists of 687 Earth days. Like Earth, Mars has its seasons, but they last twice as long. And Martian weather conditions are anything but monotonous or predictable. In 1997, when Pathfinder landed on Mars, its tiny weather station gathered data on the local Martian weather, which NASA posted on the Internet. The reports showed that temperatures range from 60° F at noon to –100° F at night. Travelers’ advisory: because of the much lower atmospheric pressure on Mars, surface temperatures differ drastically from air temperatures. If you were standing on the surface in midday, your feet would be warm and snug, but the fluids in your head would freeze. Mars’ atmosphere has fog, wind, and red dust, lending pink tints to a sky accented by two small, misshapen moons, Phobos (“fear”) and Deimos (“terror”).
Mars resembles Earth in other ways. Its polar ice caps wax and wane seasonally. There are clouds. There is ample geologic evidence that rivers once flowed freely on its surface. The stage has long been set for life to appear there. Yet the Earth teems with life, while Mars appears barren, at least on the surface. Why? No one really knows, yet the answers may lurk in the perplexing differences between the two planets.
The Earth’s surface consists of overlapping, often ill-fitting plates covering its molten interior. They form a crust like an eggshell, thin and brittle. They bump and grind against each other; occasionally they pull apart, as they are doing now in Iceland, giving rise to earthquakes and volcanoes and mountain ridges lurking beneath the oceans. Iceland sits right on the spine of the Mid-Atlantic Ridge, a segment of the Mid-Ocean Ridge, which is the longest mountain range on Earth, extending 40,000 miles, or one-and-a-half times around the planet. Iceland’s unique placement means that half of it belongs, in a geological sense, to the European continent, and half to the American. And the two halves are pulling apart at the rate of one centimeter a year. That doesn’t sound like a lot, but when this movement occurs over the course of ten or twelve million years, it eventually becomes a very big deal. Iceland could break apart and be absorbed by other, larger land masses. Or if it surges in volcanic activity, it could enlarge itself, adding enough real estate to accommodate many more hardy souls. For now, a seam runs right through Iceland, clearly marked in some places by a narrow chasm and in others by small streams and little cracks. If you jump across one of the cracks, you jump from one continent to another.
At this moment, no one knows for certain if Mars has or had plates similar to Earth’s, or, if the Red Planet did have them, how they operated. If Mars never had crustal plates, their absence poses interesting questions about how it developed without them. And if it did, we see no direct evidence of them – not yet, at any rate. The geologic processes associated with crustal plates would have affected the way life did, or did not, develop on Mars.
“Nothing you see here is more than ten thousand years old,” Jim shouts over the whine of the engine, as we pass over the Reykjanes Peninsula region of southwest Iceland, “and some of it is only five thousand years old, or less.” Jim lives by the geological clock, which extends billions of years, all the way back to the formation of the universe. The universe is an old, old place, perhaps 15 billion years old, possibly more, and the planets of our Solar System are old, too, something on the order of 4.7 billion years. When you measure time in billions of years, you dismiss a million years as a hiccup. A span of five or ten thousand years is insignificant. The concept of a year, the time it takes for the Earth to complete a revolution around the Sun, scarcely seems an adequate yardstick for measuring the development of the universe and the planets. Iceland’s arriviste status in the geological scheme of things is rare and intriguing; the place teems with clues about the formation of Earth, of Mars, and of the entire Solar System. To understand the Red Planet, even partially, is to understand something about the nature of the universe, to catch glimpses of our distant past and our future, to extend perception to a scale much larger than ordinary human comprehension, to harness the imagination to the intellect, and the intellect to the stars.
These days, planetary scientists like Jim regard the geology of Mars as crucial for understanding Earth and the other rocky planets in the Solar System – Venus and Mercury (and the moon, as well). Jim reminded me that the geologic prizes on Mars are rich. Although it is forty percent smaller than Earth, Mars’ peaks and valleys are far more extreme. The continental United States could fit nicely into one of its canyons. Its volcanoes are awesome. The largest, Olympus Mons, is more than 90,000 feet high. It would tower over Mt. Everest, and it’s large enough to occupy the state of Arizona. It is one hundred times larger than the biggest volcano on Earth; in fact, Olympus Mons is the largest mountain in the entire Solar System. Mars is a planet of geological superlatives.
Oscar levels off the Aerospatiale at 2,000 feet. Beneath us, the primeval landscape – gray and brown and black, rocky and dusty and nearly treeless – extends toward the horizon. Is this what it would be like to fly over the scarred surface of Mars? Eventually, we cross a beach, and the island of Heimaey, our stopover point, lies ahead, gradually gathering substance in the blue mist. It is a remarkably tranquil day, so calm that a limp windsock on the ground barely swivels as we veer toward the island’s tiny runway, a strip of asphalt running uphill between two volcanic peaks. Ever since leaving New York, I’ve been placing my life in the hands of complete strangers, and now, sitting beside Oscar as he casually maneuvers his small aircraft, I wonder if I’ve finally gone too far.
“Move your legs! Please!”
Oscar orders me to contract so he can freely guide us to a safe landing. The plane taxis to a standstill. We are almost there.
Jim hasn’t managed to coax NASA into funding this leg of the journey – which comes to about $300. As we slap down our plastic to pay the bill, Jim cites NASA’s “faster, better, cheaper” way of doing business to explain why we must pay the airfare to conduct scientific research. Dan Goldin, NASA’s mercurial Administrator, instituted the policy when he took over the agency in 1992. NASA, like any federal bureaucracy, has indulged in its share of waste and redundancy, and Goldin, coming out of private industry, wanted to trim the bureaucratic flab and refocus NASA. Essentially, he wanted to do more with less. He increased the number of planetary missions under the “faster-cheaper-better” regimen; instead of one expensive mission, the agency would send two, or even four cheap ones, and the returns would be correspondingly greater. And they were! But planetary exploration at any price is an exceedingly risky business, and more missions has also meant more failures. In the grip of “faster-better-cheaper,” NASA didn’t realize that the American public would fasten onto the failures of its recent missions to the Red Planet – Mars Climate Orbiter and Mars Polar Lander – and forget the successful ones. The notion that NASA was exploring the planets on the cheap and occasionally bungled the job alarmed the media, and it alarmed Congress – how could this have happened? – yet it was Congress who, year by year, imposed the budget cuts on NASA that led the agency to adopt “faster-cheaper-better.” The result is NASA Lite.
The cuts have been playing havoc with Jim’s work life. For weeks, the Iceland expedition has been in doubt because of the fragile health of the reconnaissance plane, a modified P-3. This is a large four-engine turbo-prop originally meant to fly low over the ocean to detect submarines lurking below the surface. NASA adapted this aircraft for remote sensing: measuring geological, oceanographic, and atmospheric features with instruments used in conjunction with satellites. But NASA’s P-3 is a thirty-year-old rust bucket, and it has seen hard use. Jim has reminisced about the crew’s Technicolor yawns as the plane followed the rolling terrain at a low altitude, like an airborne roller coaster. He has described the spider-web cracks that developed in the windshield during an Iceland mission in May 1996. The windshield threatened to crack wide open, jeopardizing the mission. One pilot gave an order to don emergency gear, but the other pilot disagreed, and besides, they had no emergency gear or crash helmets or parachutes. To make matters worse, they were carrying too much fuel to land, and the Icelandic government prohibits dumping fuel into the Atlantic. They had to fly for hours at slow speed, burning fuel, until they could land safely and legally. More recently, the plane developed a chronic fuel leak and lost an engine in flight over Greenland. The accumulated weight of these stories worried me. Even Jim, who does this kind of thing for a living, was anxious. I checked out the P-3 with my friend Peter, a commercial pilot who has flown all over the world in dicey equipment. Peter explained that, worst case scenario, if an engine or two quit, the plane could coast more or less gently to the ground, unlike a helicopter, which would drop from the sky. I was not completely reassured.
NASA keeps the rust bucket aloft, despite everything, “to facilitate cost-effective essential remote sensing that has inexorably been rewriting textbooks associated with atmospheric science, climate change, and the lay of the land,” as Jim puts it. In other words, this rust bucket is changing the way scientists think about how our planet works.
Despite the significance of its science missions and the public dismay when they go wrong, relentless budget cutting continues to afflict NASA. The agency now receives less than 14 billion dollars a year, less than one percent of the overall federal budget, and each year its budget shrinks a little more. The unkindest cuts of all affect people, not hardware. Dan Goldin earns about $150,000 a year, and scientists like Jim Garvin, who hold one or more advanced degrees and are often among the leading figures in their fields, earn less, something equivalent to a college professor’s salary. Unlike academics, they work six or seven days a week, year round, without sabbaticals. And NASA has stringent rules governing outside income from consulting or lecturing, so moonlighting is out of the question, even if the NASA scientists had time for such activities, which they don’t. Willingly or not, Jim and his colleagues must emulate the example of Louis Agassiz, the famous naturalist, who stated, “I cannot afford to waste my time making money.”
Why do they do it? Why do these driven scientists, who could be earning several times more than their current salaries in private industry, stick with stingy old NASA? Why do they remain oblivious to imploring spouses and former colleagues who have gone to seek their fortunes in private industry? The most these NASA scientists can reasonably hope for is recognition from their peers, if they make a major discovery. They’ll have an easier time getting grants, lots of impressive plaques to hang on the wall, and that’s about it. Despite the influence of their ideas on the course of science and exploration, obscurity is often their lot. Who can name the members of the team that in 1996 announced possible evidence of fossilized life in a Martian meteorite – a discovery that, if correct, will stand as one of the most significant breakthroughs of all time? Who can name any NASA-supported scientist, for that matter, with the possible exception of Carl Sagan? And who, outside of the scientific community, is aware of Sagan’s actual role in NASA’s exploration of space?
Sagan’s success as a popularizer of the Cosmos obscures his real achievements as a scientist, thinker, and writer. A productive scientist and winner of the Pulitzer Prize, he frequently appeared on The Tonight Show; he didn’t fit into neat categories. He was cursed with charisma. An astronomer by training, he gave a convincing impression of being at home with a number of disciplines ranging from mathematics to history. His fascination with space offered reassurance rather than terror of the unknown. He developed a benign, Jeffersonian vision of the universe as the last frontier, the ultimate, infinite West, where humanity would be able to seek refuge after fouling this planet and possibly destroying itself in the process. Sagan’s outer space, like Thomas Jefferson’s West, offered sufficient scope to alleviate humanity’s ills. He was pessimistic about the future of mankind if we were confined to Earth for too long. It seemed to him a near certainty that, sooner or later, we would blow ourselves up. The only escape from his Malthusian nihilism was the vastness of space and the promise of distant planets, where humankind could start anew. This vision of space as the new frontier influenced NASA from its inception, imparting a sense of purpose, and it inspired younger scientists by giving them a larger context for their research. In the midst of bureaucratic setbacks and budget battles, Sagan knew what was at stake in the exploration of space: over the short term, enlightenment, over the long term, the survival of humanity.
Throughout his career, he cultivated a special fascination with Mars. For him, it was a touchstone of all heavenly bodies and possibly the salvation of humanity. He wrote about it for scientists and for general readers, artfully mixing speculation and scientific fact. He prodded NASA to explore. And he held out hope for life on Mars. As early as 1966, when the conventional wisdom in the scientific community, chastened by the barren photographs resulting from the Mariner missions, held the chance of life on Mars to be zero, Sagan, almost alone among prominent scientists, speculated that such a phenomenon might still be possible.
Sagan influenced a generation of younger scientists, who have their hands on the levers of the future and who fervently believe that now is their time to change scientific thinking about the nature of the universe and our place in it. They stick with their work for many reasons: because they can’t do without it; because NASA gives them the means to do what they’ve yearned for since they were children growing up in the heyday of the space race, watching John Glenn go into orbit; because NASA will let them send something of their own design – a part of them – into space; because NASA has the rockets and the launch facilities and the infrastructure to get it done; because NASA will validate their work in the eyes of the scientific community and the world. Because, when it comes to planetary exploration, NASA is the only game in town.
The little airport on Heimaey is deserted; the Iceland Coast Guard helicopter has yet to arrive. Oscar sits at a table in an empty café, smoking a cigarette. Jim, wired, munches on a Mars bar (“It’s my planet, Larry. I may as well”) and reminds me that twenty-five years ago, this quaint little island (“Heimaey” means “Home Island”) had to be evacuated when that volcano – over there – erupted, and lava poured down its slopes into the village. When the eruption ended, Eldfell, as the volcano is known, had transformed the island. It was fifteen percent larger and contained thirty million additional tons of lava, which the local populace later used for roads and buildings. Nor was that eruption unusual for Iceland. Every five years, Iceland witnesses a major volcanic eruption, some capable of sending enough ash into the atmosphere to darken the hemisphere’s skies and lower global temperatures. In 1783, the largest volcanic eruption observed in modern times occurred in Iceland. It lasted for months and disgorged over two hundred square miles of lava. The explosion hurled sulfur dioxide particles into the lower atmosphere; they in turn caused acid rain that polluted the ground, poisoned cattle, starved a quarter of Iceland’s population, and darkened the skies over Europe. (A natural catastrophe of that magnitude has undoubtedly occurred on Mars.) Iceland is overdue for another eruption, Jim remarks casually, and an active volcano dominates the island where we will spend the day. The island’s name is Surtsey.
The newest place on Earth, Surtsey is even more Mars-like than the rest of Iceland. It was formed in a mammoth undersea volcanic eruption that lasted from 1963 until 1967 and, during its early phases, lit up the night skies for miles around. It was named for Surtur, the fire-bearing giant of Norse mythology. The island is younger than Jim, who was seven years old when it erupted into being. At the time, his family was living in Beirut, where his father worked for IBM. Jim’s maternal grandmother, who was living with them, became fascinated by the eruption. She collected all the newspaper clippings about it she could find and showed them to her grandson, giving an unexpected direction to his life. Jim has remained in Surtsey’s thrall ever since. The vanity plates on his ten-year-old Jeep announce, “SURTSEY.”
Only a couple of hundred people have ever set foot on the island. Access is extremely difficult. The North Atlantic currents surrounding it are too rough for most boats to negotiate; swells around the island are frequently twenty feet; waves have been said to reach eighty feet. The island is off limits to everyone but a few heavily credentialed scientists who have obtained permission from the Icelandic government to conduct research there on a “non-biologically interfering basis.” In practice, this restriction has made Surtsey into one of the largest and most carefully studied natural laboratories on the face of the Earth, of interest to geologists because of its recent, well-documented formation; to botanists and biologists, who track the development of life; and to Marsists like Jim Garvin who regard a visit to Surtsey as the closest they’ll ever come to the Red Planet.
The only practical way to reach the island is by helicopter, weather permitting. “There are no guarantees, as helicopters only go out that far once a month,” Jim alerted me several weeks earlier, when we were starting to get serious about the field trip. “Please note there are NO insurance provisions. ANYONE going to Surtsey does so at his or her own risk, and there is some, as the island is still HOT and there are hydrothermal systems with 120° C water just beneath the ground. Also, the weather can change, and people have been stranded. I was urged to remind you of this. Also, what I must do out there will require vigorous hiking over lava and volcanic ash. Anyway, there is a chance we will get there for a day that I do believe you will thoroughly enjoy.”
Jim once mentioned to me that his colleagues considered him a bit eccentric. After pondering his disclaimers and warnings concerning Surtsey, I recalled a strange story I’d heard about him. In the heyday of the Apollo program, NASA was thinking seriously about sending people to Mars, yet the agency hesitated. Guiding a robotic spacecraft to the Red Planet is an intricate, ambitious, and unpredictable undertaking; a human mission would be far more risky and complex. NASA was stymied by the problem of getting its astronauts home. Jim came up with a unique solution: he offered to go to Mars on a one-way basis.
When I asked Jim about this story, he was mildly abashed. “I’m not proud of this now, but when I was younger, before I married and had kids, I volunteered to go.”
“One way?”
“Well, yes. The only way you could get a man there was one way. It would be too costly to get him back to Earth. So I would go there, have enough life support to explore and survive for two years, and then …”
“And then?”
“That would be all. And it would have been worth it, the scientific returns would have been spectacular, but that was before I had kids. Now I have other responsibilities I didn’t have then.”
The thin tin walls of the Heimaey airport terminal start to vibrate. There’s a sound almost below the threshold of hearing. We feel it in our guts as it gets louder and more intense. Thwacka-thwacka-thwacka … Rotors whirring, the Iceland Coast Guard helicopter, an impressively large and sturdy Bell Jet Ranger, descends into view. It is a noisy piece of equipment, manned by a crew outfitted with Day-Glo orange flight suits. These are the men of the Iceland Coast Guard, and they swarm around us like giant orange insects. A small group of Icelandic botanists has joined us, and our little group approaches the helicopter, deafened by the whine of the motor and the thumping of the rotor. A crew member, smiling crazily, hands each of us a life vest and a helmet equipped with a microphone. I place the helmet snugly over my head, and the unbearable thwacka-thwacka-thwacka subsides to a distant drumbeat. We strap ourselves into the seats, and the helicopter slowly rises from tarmac to a height of about six feet. We delicately revolve until the nose suddenly pitches forward, and we take off like a shot. This is flying. We swoop over the ocean at an altitude of about 500 feet, until we reach the island of Surtsey, fifty miles from nowhere. From the air, the place looks so barren and primitive and devoid of anything recognizable that even Heimaey, by comparison, seems civilized. The jagged gray lava formations of Surtsey rise to greet us. The helicopter sets down lightly on a concrete landing pad considerably smaller than my living room.
Less than twelve hours before, I was sitting in the back of a taxi cab in New York City, and Jim was fighting traffic on the way to Baltimore-Washington International Airport. It is now 10:30 AM local time on a rare, beautiful morning on sub-arctic Surtsey. Our coordinates are 63° 13” North, 20° 31” West.
The rotors slow almost to a stop. We emerge from the helicopter and wave merrily to the crew. The helicopter begins to whine, the rotors fling gritty volcanic ash into the air, and the machine lifts off.
It tilts toward the mainland and disappears, leaving a therapeutic silence.
We are alone on the newest place on Earth.
The temperature is in the high fifties, about as warm as it gets on the surface of Mars. True, the atmosphere here is saturated with oxygen and the gravity is approximately two-and-a-half times greater than the Red Planet’s, but as far as Jim is concerned, he’s on Mars.
Jim believes this is a particularly auspicious day to begin his mission, since July 20 is “Space Exploration Day,” also known as “Moon and Mars Day.” On this date in 1969, the Apollo 11 astronauts stepped onto the lunar surface. Seven years later to the day, the Viking 1 lander transmitted the first images of the plains of Chryse to Earth. Quite a date, when it comes to planetary exploration. Some day, he believes, July 20 will be designated a national holiday for space exploration.
The botanists disperse ahead of us. Although he is exhausted from a sleepless night, Jim heads out with a bundle of topographic maps under one arm and a pair of bright orange Swarovski laser binoculars around his neck. “They are good to three-tenths of a centimeter. I have about fifteen different programs I can set it on, and if I want, I can connect it to my laptop,” he says. He wears hiking shoes designed for walking across lava, an olive drab NASA flight jacket, and a white baseball cap bearing the legend “Mars Observer,” a reference to the billion-dollar NASA spacecraft that vanished in 1993. No trace of it has ever been found, nor has a wholly satisfactory explanation been offered for its disappearance. Jim refers to the incident as an “act of God,” and wears this cap as a casual memorial to the lost mission.
As he sets out on this glorious Surtsey morning, he experiences an overwhelming sense of déjà vu. The last time he was here was 1991, and now he suddenly feels as though he’s home again, and he wipes his eyes. There’s a spiritual dimension to his scientific exploration that he can’t quite put into words. It’s an epiphany – a scientific epiphany, if there can be such a thing. “I felt as if I were back at a good place for learning and experiencing how life gains a foothold on a previously unborn, sterile world,” he later told me, when he was better able to verbalize, but now, at the moment he sets out, it’s the adrenalin rush he’s feeling, the intoxicating sense that the world, or this little volcanic part of it, anyway, belongs to him for the time being. There’s so much going on in his mind – calculations, memories, nascent hypotheses – that I think of Garvin as a highly emotional computer.
He allows the nostalgia to wash over him, and goes to work. Although Surtsey looks stonily barren from the air, close inspection yields different results. “Observing change is the central theme of Earth and planetary science,” Jim tells me. “What is changing? What has changed? Has life on the Red Planet – if it’s there, that is – changed or been changed by Martian conditions over time? Have environments on Mars eradicated the footholds of life that may have been established at one or more times in Martian history? With Surtsey, I am struck by the incredible pace of biological change in only seven years. What appeared as a nearly sterile, Mars-like vista in 1991 is now an alien landscape complete with kitchen-table-sized mounds of flourishing higher-order plants, dunes covered by grasses.” Jim mentions that ecological succession has changed the face of Surtsey at a rate ten, or even a hundred, times faster than typical terrestrial landscapes, and perhaps a million times faster than change on Mars. Thanks to the action of wind and water, Surtsey is destined to vanish beneath the surface of the ocean almost as quickly as it arose. “I’m going to have an equation by October that will contain the predictive lifetime for Surtsey,” he says. “We’ll have the first landscape volume erosion rate for an isolated volcanic island.” If the present erosion rates hold, Jim predicts Surtsey will vanish beneath the waters of the North Atlantic by 2045, and his subsequent calculations confirm this date. Think of this process as geological time-lapse photography. A world arises and vanishes before your eyes.
Ahead lies an impossibly steep curving wall of solid rock, 460 feet high; to the left, a craggy, broken crater – a tephra ring about fifty yards across. A tephra ring is a partial crater formed as exploding volcanic ash falls to the ground in a semicircle, usually molded by the prevailing winds, which on Surtsey can be fierce. Geology-speak is a Babel of languages. Tephra is Greek for ash. Lava is Hawaiian. Many other terms are Icelandic, which is among the oldest continuously spoken languages in the West, the language of the Sagas, and, at times, the language of geology.
Lava, in Icelandic, is hraun. “It’s the oldest word for lava there is,” Jim says. There are several subsets of hraun: apalhraun, which is rough lava, and helluhraun, which is smooth. A small volcano in Icelandic is a dyngja. Hlaup means “flood,” and jökull means “glacier.” If you put those two words together – jökulhlaup – you get something for which there is no exact equivalent in English: a catastrophic outburst flood caused by water trapped under a glacier, which cracks open the ice and violently disgorges.
This catastrophic event occurred in 1993 on an Icelandic flood plain called the Skeidararsandur. Blocks of ice as large as houses tumbled for miles across the flooded black primeval landscape in an orgy of geologic violence. A similar geological disaster also occurred on Mars in the distant past. The scale was immense. It is estimated that the Martian jökulhlaup released as much as 100,000 cubic meters of water per second, more than the entire flow of the Amazon river.
At the moment, we are standing on hraun, or, more precisely, helluhraun, with a little apalhraun scattered here and there. Looking into the tephra ring, Jim says he’s stunned “to observe the development of erosional canyons massive enough to drive a Hummer through.” He didn’t see anything like this on his last trip to Surtsey. The erosional scars remind him of features shown in the latest images from Mars Orbiter Camera, now circling the Red Planet.
“These mini-canyons, technically erosional gullies, expose the underbelly of Surtsey, the volcano. They give clues about its future and the processes that formed it. The sheer beauty of these signs of geologic aging and their abundance are remarkable!” He takes a closer look at the black windblown tephra. “See how it’s sorted? See how the small rocks have risen to the top? That sorting is common. Some of them are rounded.” Those smooth contours, he tells me, are diagnostic of wind and water, and he looks for similar shapes on Mars. “So far, we haven’t found a lot of really rounded ones on Mars,” he admits. But he keeps looking because evidence of water is essential to the detection of life beyond Earth. In fact, water has assumed such importance that the question of extraterrestrial life has been reframed; where scientists once inquired, “Is there life elsewhere in the universe?” they now ask, “Is there liquid water elsewhere in the universe?”
Many planetary geologists, Garvin included, now see convincing evidence that Mars once had lots of water, and may still have a tremendous amount of water even now. Their goal is to follow the water because they hope it will lead them to life. So they seek distinctive water signatures. They look for evidence of dried-up rivers and oceans and shorelines; they theorize about subsurface water, and they measure glaciers – anything associated with water.
Stepping lightly on tephra, Jim makes his way across the eastern side of the island, squinting and kneeling, taking measurements, orienting and reorienting himself, studying the landscape, observing the reverse sorting of the soil, in which “coarser fragments the size of popcorn nubs rise to the top of the soil horizon, leaving the finer, claylike fraction below.” He notes the fragmentation of large blocks of volcanic rocks. On the right, Jim confronts a landscape studded with pitted blocks ranging in size from softballs to basketballs. Those pits grab his attention. He spent a good deal of his graduate career at Brown in the mid-1970s studying patterns of pits and surface textures on terrestrial rocks and on Martian boulders photographed by the two Viking landers, trying, as he put it, “to unravel the geologic secrets of Mars.” Here is a banquet of strikingly similar boulders, on which he is ready to feast. He notes unpitted gray rocks with angular shapes – so-called “country rocks” – as well as pitted rocks, whose morphology speaks to him, telling of displacement from a lava flow.
Jim explains how this local landscape came to be: “Once the sea water was kept out of where the lava was bubbling up, a carapace of lava formed. And that lava is very important, because it protects the vent. The vent is where the hot rock comes up. That is the reason this island survives today.” He displays what looks to me like an ordinary rock, but to Jim, it’s a geologic sonnet. “This is tephra that tumbled downhill. See how it’s made up of bits of other stuff? It’s actually a breccia. A breccia is stuff made of other stuff, little welded bits as strong as concrete.” As I hold the raw geological material in my hand, Jim reminds me that this is what the rocks on Mars look like; the main difference is that they’re coated with a brown dust. He feels around the edges. “It’s a smooth little rock,” he says. “That means it’s been worn by erosive agents, so we look at the rounding of the corners to get an indication of what’s going on.” I carefully replace the rock so as not to disturb the course of Surtsey’s geological evolution.
The hraun we traverse feels like soft beach sand. Jim tells me that on Mars, the soil is ten times finer than what we’re walking on now. “It would be more like walking on talcum powder.”
We press on, and the terrain subtly shifts. “Now we have coarse stuff lying on the surface,” Jim remarks, as he tries to read the landscape. “Here’s a little piece of basaltic pumice. That’s a good one,” he says, slipping it into his pocket, which is, perhaps, not quite kosher. “That’s one for the spectrometer,” he explains. There’s an honor system in force here. You’re not supposed to disturb anything. You try not to leave footprints in this haven for scientists if you can possibly avoid it. “Now, this looks like – aha! This –” he announces, “is a little lava bomb.”
“What?”
“A lava bomb is something that flew through the air and went splat! And then it started to break. Already, it’s weathering away. See how it’s crumbling. Again, this is what we looked for at the Pathfinder landing site.” He calls my attention to smooth rocks inside smooth rocks, and he begins to interpret. “You can piece together the history of this rock,” he says. “These rocks were always smooth; they got pasted together at the time of the eruption.”
He sees some similarities between the geology underfoot and the Pathfinder landing site on Mars. NASA sent Pathfinder to a location on Mars where it was believed that a great outpouring of water once occurred. “Some people think the rocks in Pathfinder’s vicinity came to rest there as the result of one big flood, but that’s ludicrous. It’s a mixed population of rocks around Pathfinder,” which suggests, to him, at any rate, that the geological history of the area has been fairly complex. Water might have come and gone around the Pathfinder site more than once over the eons. I look around; if you photographed a replica of Pathfinder here on Surtsey, you could persuade a fair number of people that the spacecraft was actually on Mars. The more Jim talks, the more I feel a geological kinship between the two planets; Mars seems so Earth-like, or is it more accurate to say that Earth is so Mars-like?
Garvin kneels to inspect a delicate lava formation. “See the thin carapace of lava? This black stuff?”
“It’s very soft.”
“Right, very soft underneath.”
“It’s falling apart.”
“Not all of it. And that’s important, because that’s the action of a process that tears down rocks and makes clays. We take clays for granted. On Mars, there’s likely to be a lot of clay.”
“And water is necessary for clay.”
“Yes. You have to break rocks. Look at this.” He points to where the hillside is collapsing. “What you see is little mudflows. And look at this. Here is a beautiful little lava rock! Very angular. This is a classic, coated with fine-grain stuff. It’s almost a pentagon.”
Jim points to the volcano’s peak looming overhead and recollects the last time he climbed it. “The wind was blowing at forty-five miles per hour the whole time, and it was very hard even to talk.” That windspeed was moderate, by Surtsey standards; the island endures 200 days of gale-force winds a year. “When we were here in ’91, this area was a desert, but plants are taking over now.” Now, the main plant in evidence is the lowly sandwort, a simple succulent that has proliferated on Surtsey with astonishing speed; small, dense, and tenacious, it can boldly go where other vegetation can’t. Even mosses can’t get a grip on Surtsey; the wind rips them out of the ground and flings them away.
“Look! There is a gorgeous breccia. Notice it’s in a little hollow, okay? That’s called an apron. We look for those kinds of things on Mars. Outside, you can see there’s a layering to it that’s caving in. See the carapace of lava up there? It’s starting to break off. In a big storm, that could fall.” It looks like the burned crust of a pie at the edge of a pan. “Now, see how these rocks are perched? Notice the pits. That’s where Mars comes in.” You see something similar in images from Pathfinder, Garvin says – pits left by primary gas bubbles in the lava. He snaps a picture of the pitted rocks on Surtsey as he continues. “Look at these pitting textures! All different. It’s exquisite.”
He zeroes in on a block of lava that speaks to him in a private language. Crouching, he declares, “Now, this is not primary lava. It’s softer, and it’s been coated with a bright alteration stain caused by chemical weathering. It’s allochthonous. That means it’s out of place, been moved away.” I step back to take in the scene, and I realize the site looks like the Grand Canyon in miniature. “This could be the beginnings of a little Martian canyon system,” Garvin exults. “It’s gorgeous. Oh, God, wouldn’t I love to measure that with a laser!”
We’ve been picking our way around the base of the volcano, and now we turn away from it and face the ocean. Before long, Jim again shouts. “Look at that.” He points to a slight discoloration on a mound of stones, in which he sees vast implications. “That’s the high water mark from a wave, where the fine dust coated the rocks. Now that is the kind of shoreline we are looking for on Mars.” The subject of ancient shorelines on Mars carries the charge of controversy and borderline heresy. Several scientists have tried mapping the shorelines of ancient Martian oceans that vanished a billion or more years ago, but their work has yet to gain widespread acceptance. I try to imagine Mars as a wet place, covered with oceans, teeming with possibilities, but this is like trying to visualize oceans in the Sahara, for Mars is red and dry and cold.
A large empty plastic bottle catches my eye, disturbing my reverie. The object seems as incongruous here as it would on the surface of Mars. We notice pieces of plastic, and buoys, and rope, and blocks of wood studded with rusty nails. “The garbage of humanity,” as Jim calls it, has drifted out here, fifty miles from nowhere, a mocking reminder of home. All day long, he has been scrupulous about not disturbing plants or lava or rocks, to the point of walking in old footprints. Avoiding the detritus, we cross a hard, crusty portion of the beach. “Hard pan clays,” he remarks. “See how they crack? They’re desiccated. We look for things like that on Mars. More indirect evidence of water. Here on Surtsey, we have a microcosm. We have a scale where it’s easier to see things. One of the things about Mars to remember is that it’s a big planet, about forty percent as large as Earth. If we land in three or four places on Mars, we’ll learn about them, but we won’t get the big picture of Mars that way, so we study sites on Earth that we believe operate in a similar way.”
We approach the water’s edge, but a formidable barrier repels us: a giant collection of round, basketball-sized rocks. “If we ever saw a field of dense, interconnecting rocks like this on Mars, we’d know the action of water was responsible. But, we haven’t seen this, yet.” As a geologist, Jim looks for patterns, distributions, colors, textures, and shapes. He is the detective, and they are his fingerprints. If he successfully unravels the geological mysteries of Surtsey with them, he will also know more about the development of the Red Planet.
Turning away from the beach, Jim and I finally begin the ascent to the volcano’s summit. I’ve been trying to put off this chore, but here it is, the thing we must do. Jim reminds me that we are climbing an active volcano, and there’s always a chance that it could blow without warning. I recall Iceland’s uninterrupted pattern of volcanic outbursts every five years for the last 1,100 years, and I remind myself that it’s due for another eruption. I feel as though we’re crawling up the side of a giant, overstressed pressure cooker. Jim tells me that a series of sensitive seismometers has been placed on the volcano; in fact, all the volcanoes in Iceland are similarly equipped, and the seismometers are so sensitive that they can detect microseizures involving magma, or molten rock. I’m somewhat relieved to hear about this detection system, but in the event of a warning, I wonder how anyone would be able to convey the news to us. Six months after our visit, a big volcano finally did erupt beneath Iceland’s largest glacier, Vatnajökull, located on the southeast coast, home to most of the country’s population.
The gray lava and rounded rocks give way to a smooth, steep incline. Jim estimates it’s twenty degrees, but it feels more like thirty to me, very steep, indeed. We zig-zag our way across, and look down on the larger of Surtsey’s craters, a craggy rusty red configuration filled with volcanic ash that from this height resembles a soft, inviting mattress. The wind picks up, and we crouch to avoid being flung down the slippery side of the mountain. Wind, incidentally, figures prominently in the Martian environment. On the surface, dust devils are everywhere. In the upper atmosphere, winds can reach 350 miles per hour, and wind storms occasionally engulf the entire planet, obscuring the surface for days.
Jim reaches a seep, a place where the ground comes apart, as if it were fabric that has been rent. A faint plume of steam rises from the wound, and the smell of sulfur permeates the air. Kneeling beside this smoldering, malodorous seep, I begin to think of Hell as a realistic notion, based on observable geology. Jim asks me to place my hand on the soil near the edge, and it feels like hot clay. A fine white crust along the rim contains bacteria that thrive in the heat and sulfur. This is the most primitive type of life on Earth, Jim reminds me. Life may have begun in volcanic seeps similar to the one at our feet, and it might have started the same way on Mars, on other planets, and on countless moons and asteroids – if it ever did.
These bacteria are examples of extremophile life, primitive life forms that have recently been discovered in places where biologists once assumed life could not survive because the conditions were too hostile – too hot, too cold, too dark, too salty, too deep. In recent years, many of the assumptions about the requirements for life on Earth – and, by implication, the possibility of life on Mars and other celestial bodies – have been overturned.
“We are finding out about the tenacity of life,” Jim said before the trip, “and it’s startling. We’re finding creatures that live at five times atmospheric pressure two miles deep in the ocean in places where the water would boil if there weren’t tons of pressure on top of it. We’re finding giant simple worms that look like garden hoses that live under those conditions. They don’t need any light, they scavenge the sulfur produced in volcanic eruptions deep in the ocean. They live off sulfur; they eat bacteria that grow in the sulfur, and that sustains them. Is there sulfur on Mars? Likely.” Life flourishes just about anywhere, it turns out, no matter how extreme the conditions. “Can you stick life a mile down in rocks and have it survive and bloom? Yes. Can you put it two miles deep in the ocean where there is no light of day, ever? Yes. Stick it on the coldest place on the planet and it will at least remain dormant there? Yes! Now, if you can form niches of life on Earth in such horrid environments, with pressure that would crush a human being to pulp and temperatures that would boil our skin – if you have life forms under those conditions, then it gets quite interesting. In fact, the question now in biology is: can you even produce a sterile environment?”
The question got me thinking about the famous Miller-Urey experiment designed to illuminate the origins of life. In 1953, two scientists at the University of Chicago, Stanley L. Miller and Harold Urey, put gaseous methane, ammonia, water vapor, and liquid water – ingredients thought to simulate a primitive Earth atmosphere – into a closed system, and sent an electrical discharge spark through the mixture. The gases interacted, and a gummy residue formed; analysis showed it contained organic molecules, including many amino acids, which are the building blocks of life. It had been previously thought that the prerequisites for life were rather special and demanding and occurred only on Earth, but the experiment suggested that all you needed to produce life were a few simple, readily available chemicals and an energy source. These things could be found on other planets, on some asteroids, and most probably on Mars. You don’t need oxygen for life to develop, and you don’t even need the Sun; the heat source could be volcanic or subterranean. I asked Jim, “If you put together all the necessary ingredients, does life inevitably develop?” Because if it did, it could be developing on Mars and throughout the universe, wherever those things are found.
“Larry,” he said, “you’ve just asked the Genesis Question. We don’t know the answer. Some people believe it could, some believe it couldn’t. A few billion years ago in the history of this planet, and in the history of Mars, and possibly in the history of other places, there may have been very sporadic conditions that might have been able to sustain life. But that was at the time when the planets were being constantly bombarded by junk leftover from when the Solar System formed. There was a lot of leftover crap, and it eventually smashed into the planets. We think all the planets formed about four point seven billion years ago in a relatively commonplace little spinning nebula of dust that collapsed to produce them and also spun off stuff that didn’t quite make it, like the materials in the asteroid belt that occasionally crash into us.” And then he said: “There was even an idea that life sprang forth on those objects, and there was a great so-called ‘panspermia’ wherein life spread from one place to another from some unknown source. Not us. We weren’t the source, according to panspermia theory. We were just one of the places where it landed and survived.”
I casually remarked that panspermia sounded like the answer to the question of life in the universe.
“Be careful,” Jim said. “The idea is very controversial, and often misunderstood. A lot depends on whom you talk to.” Although there is no consensus about life on Mars now, he told me, many scientists have come to believe that it’s very hard to imagine that Mars didn’t have a failed attempt at life forms at some point in its history. “The question is: where did it go? Seeing the existence of life on Mars would be like finding the Rosetta Stone. We may be alone now, but not in the past.” Jim thinks of Mars as the mother of all control experiments. “The theory goes like this: the Earth is a very messy, complicated, intersecting set of systems, but we also need a sandbox to play in, and the best sandbox we have is Mars. It’s a natural control experiment for things we want to understand about our own planet, if we were able to strip away and isolate some of the variables. For instance, Mars is colder and drier. Water exists there as ice or as a gas in the atmosphere. When it did exist as a liquid, it probably did so only briefly. There is no biosphere altering the planet, as we have on Earth. If it ever started, it failed.”
It’s possible that we could end up like Mars, as the Sun fades. Jim tells me that if all the water on Earth froze and then evaporated, we could very well have conditions that would suck the oxygen out of our atmosphere without renewing it.
I begin to think of Mars as Earth reduced to the essentials. For purposes of scientific research, it’s more promising than the moon, even though it is much harder to reach. “Back in the days of Apollo, we could use military-class technology to zip up to the moon and fly around and be very clever because we had unlimited funds and a national commitment from our president to put human beings there. We don’t have that commitment for Mars,” Jim reminds me, making the idea of regular transits to Mars suddenly sound sensible. “People argue that NASA will never have carte blanche like that again. Nowadays, you have to keep the price way down. It means that when you go to Mars you can’t carry enough fuel to go into the orbit you want. You have to use the gravity and atmosphere of Mars itself to get you there.”
Jim takes heart from historical precedents for these difficulties. “Think of them in terms of the exploration of our own planet,” he says. “Think of the early sailors willing to risk their lives sailing from Greece to Crete, an island about a day away, if the wind blows right. They might be willing to do that because, what the heck? That’s analogous to going to the moon, which we can reach in a matter of a few days. Now imagine sailing not from Greece to Crete but from Greece to North America. That’s the scale of difference we’re talking about when we send spacecraft out to Mars.” At that scale, the celestial sailors will have to learn to improvise in order to survive, just their maritime forebears did.
While we linger at the seep, Jim reminds me that only thirty-five years ago, there was nothing here but the Atlantic Ocean and fresh air. And now we are standing on rock containing copious evidence of bacteria. Could life have spread as quickly on a Martian volcano? Well, why not? No one knows. Questions like these form the basis for “astrobiology,” the search for extraterrestrial life – generally in the form of primitive bacteria invisible to the naked eye. Although the questions posed by astrobiology – or, as it is sometimes called, exobiology – have concerned NASA scientists for over twenty years, the field has suddenly entered a period of rapid expansion, as it moves from the realm of the purely speculative to the potentially demonstrable.
Biologists are coming around to the idea that Earth, while complex and idiosyncratic, is hardly unique. Our planet does not necessarily contain a divine, magical, or fluke recipe for life. On the contrary, life emerged here when our planet was less than a billion years old, as the outcome of geologic and chemical processes. It might have been the inevitable outcome; if so, it could easily appear throughout the Solar System and the universe.
In that case, why has extraterrestrial life been so hard to find? One thing is now clear to many scientists. As the song goes, they’ve been looking for life in all the wrong places – mainly in moderate, sunlit, moist environments. As biologists develop a greater understanding of all the unlikely, remote places where life exists on Earth, it has become apparent that there is much greater latitude. Life forms can be so hardy and unpredictable that they will find a way to exist just about anywhere. And at the microbial level, life can be so simple it seems barely alive at all. Still, to qualify as life, the stuff has to satisfy at least two widely accepted conditions. It must be able to replicate, and it must be able to mutate and evolve. Darwin’s principles of natural selection apply at all levels of life, and if life is discovered on Mars, or anywhere else in the universe, natural selection will apply there, as well.
We make our way along shallow erosional gullies, which provide a foothold on the volcano’s sheer upper reaches, until we arrive at the summit of Surtsey, a precarious location high above the surface of the North Atlantic. Jim, who’s lighter and more agile, is a lot better adapted to climbing than I. The jet lag and lack of sleep are taking their toll; my heart thumps wildly, and the wind pushes me off balance. I look up, trying to orient myself. Heimaey, so solid and inviting by comparison, floats in the distance, and beyond, Iceland itself. After a brief rest, we head down the steep slope.
By mid-afternoon, we reach a small research hut at the base of the volcano, where the Icelandic botanists who flew in with us have gathered. A pot of water comes to a boil on a little propane stove, a welcome sight, a bit of Earth on Mars. Over a mug of instant coffee, I converse with a botanist, Sturla Fridricksson, who, Jim explains, is considered the grand old man of Surtsey research. Sturla’s face has been seamed and cured to a leathery perfection by the Northern sun. He looks as though he’s served time on the Kon-Tiki. Just as he launches into a complete geological history of Surtsey, a saga in itself, the Icelandic Coast Guard returns to rescue us. Their helicopter touches down with a great throbbing racket; the rotors feel like they’re sucking the air right out of my nostrils. Silent and overwhelmed with impressions from our day’s exploration, Jim and I begin the journey back to the mainland, as though returning to Earth.
When he’s not climbing active volcanoes, Jim Garvin often roams the hallways at his place of work, NASA’s Goddard Space Flight Center in Greenbelt, Maryland. That was where we met, exactly one year earlier, when I was visiting a friend who also works there. Jim was standing in a busy corridor, holding forth on the subject of Mars, and within minutes, the sound of his voice attracted a crowd of curious scientists, who drifted away from whatever they were doing to listen. Somebody ought to be getting this down, I thought, and started to take notes as fast as I could. When we began to talk, he identified himself as a co-investigator for the Mars Global Surveyor (MGS), a state-of-the-art spacecraft designed to orbit Mars and conduct a number of pioneering experiments, including mapping the surface of the Red Planet in more detail than is available for Earth.
His special area of interest, he explained, is an instrument on MGS known as a laser altimeter – a laser designed to fire impulses at the surface of Mars. Minute fluctuations in the time it takes for the impulses to return create a three-dimensional picture of the surface, accurate to within a few meters. This is an incredibly intricate engineering feat – akin to extending a tape measure all the way from New York City to Washington, D.C., to determine the surface variations on the dome of the Capitol, while recording the results in a moving car back in New York.
At that first encounter, Jim invited me – as he does everyone he meets – to share his obsession with Mars. He is a rigorous scientist, but underneath the rigor lurks a romantic explorer. Mars is not just a planet to him; it holds, potentially, the answers to the riddles of the universe. At the time of this meeting, in July 1997, the Pathfinder spacecraft had just landed on the Red Planet, and its tiny rover, Sojourner Truth, had captured the imagination of the scientific community and people around the world, who were able to follow the extraterrestrial proceedings closely on the Internet. As I talked with Jim about the development of Mars exploration, it occurred to me that Pathfinder belonged to a much larger story – mankind’s exploration of Mars – and that the exploration was itself part of an even larger story: the search for the origins of life on Earth and throughout the universe.
Despite the sophistication of the new missions to Mars, Jim waxes nostalgic about the Viking program of the mid-70s – “the Cadillac of missions,” he says. “They actually had better equipment then.” Of course, it cost the American taxpayer about ten times as much as the current hardware does. He became involved with the Viking missions when he was still an undergraduate at Brown; a geology major, he helped to analyze images from the Viking 2 lander spacecraft, and he got hooked on the study of Mars. (Planetary spacecraft come in three basic varieties – flybys, landers, and orbiters. The flybys whiz past a planet on their way to somewhere else. An orbiter circles a planet. And a lander touches down on the surface.)
Just when he thought he’d found his vocation, the Viking missions ended, and NASA closed the book on Mars exploration. The missions, Jim often says, were the victims of their own success. They sent back thousands of stunning color images, and provided enough data to keep scientists occupied for two decades. They accomplished so much it seemed there was nothing left to do except send people to Mars, and there wasn’t enough money in the budget for that.
After graduation, Jim went to Stanford for an advanced degree in computer science. The life of a geek was not his style. So what if he could de-bug his colleagues’ programs and make them run faster? The work was too routine, too solitary, too stationary. He returned to Brown for his Ph.D. in geology, where he studied under Tim Mutch and Jim Head, who also taught a popular undergraduate course known as “Rocks for Jocks.” One day, Mutch said to Head, “You know, there are no fundamental problems left on Earth.” Mutch turned his attention to the planets and published an important – one is tempted to call it groundbreaking – book, The Geology of Mars, in 1976. This was a revolutionary idea, to study the geology of the Red Planet in a scientific manner. Geology claimed a gigantic new turf: the Solar System, and, by extension, planets and asteroids everywhere. All at once, geology became an integral part of the exploration of space, and Mutch was leading the way, training a new generation of planetary geologists, including Jim Garvin.
“At first glance,” Jim says, “Tim Mutch might have been perceived as a Jimmy Stewart type of character: tall, thin, amiable, and always above-board, almost self-deprecating. Deeper inside the man was his passion and resolve.” Occasionally, he’d remark to Jim, in an offhand way, “You’re a Mars person. Did you know that?” And at a party, he buttonholed his fast-talking young graduate student and said, “Jim, you and a few others are the future of Mars exploration, so it is yours to make it happen.” That was, he says, “heavy stuff” for a twenty-one-year-old grad student to hear.
As it happened, Brown played a role in analyzing data from the two Viking landers, so Jim had access to the latest developments in Mars research and analysis. He still revels in the memory as if it were his first love. It was his first love. In defiance of conventional geological practice, Mutch concentrated on the enigmatic landforms of Mars. “This was revolutionary thinking to me, as most geologists argue that studying typical landforms is the best way to learn how a surface was formed,” Jim says. “But Tim argued that finding those enigmatic landscapes might be more pivotal in the workings of Mars than background normal landscapes.”
In 1980, Tim Mutch led an expedition to the Himalayas. He made a successful ascent accompanied by two graduate students, but the weather turned foul during their descent. One of his crampons broke, and it was impossible for him to continue. The students wanted to carry him down, but he told them, “No way. Strap me in here. Go back to base camp and get help and come back for me.” By then, he might have been delirious from lack of oxygen. The students went down to base camp, and he probably thought they’d return in an hour to rescue him, but they had a rough time getting through the storm, and by the time they made it back, eight hours had passed, and there was no sign of Tim. His body was never found. The best guess is that the storm blew him off the mountain.
About a year later, Tim’s widow, Madeline, held a memorial gathering to which Jim was invited. She showed slides taken during her husband’s fatal descent. It was unbearably moving, especially for Jim, who had been Tim Mutch’s last graduate student. In an obscure but deeply felt way, Jim believed that as Mutch’s disciple, he was supposed to carry the torch – but where? He didn’t know, and even today, he still doesn’t know where, exactly, but he always hears Mutch’s voice in his ear, pointing the way to the Red Planet. And NASA was the only way to get there.
During Jim’s early career at the agency, an unofficial Mars Underground developed within NASA’s bureaucracy. This was a loosely-knit affiliation of scientists and engineers who maintained a keen interest in Mars, despite the agency’s lack of Mars programs, and who also maintained a fervent desire to return to the Red Planet, first with robotic spacecraft, and later, with people, if the money and the motivation could somehow be found. The Mars Underground published papers, held symposia, and tended the flame through difficult times.
These were not easy years for Jim. An instrument he’d proposed, a radar altimeter, was initially selected for a Mars mission, but later deselected, or dropped. Soon after, in January 1986, the Space Shuttle Challenger disaster threw the agency into crisis. A period of soul-searching ensued within NASA. He worked for Sally Ride, the astronaut, on a project designed to renew the agency. Out of copious discussions, the Ride committee produced a grand new vision for NASA: the United States must return to the moon, and, beyond that, establish a permanent lunar base. Their recommendations were never acted on. After the group disbanded, Jim’s laser altimeter was selected for the Mars Observer mission, which ended in catastrophe in September 1993.
Finally, in 1996, Mars’s time came round again. First, there was NASA’s announcement of the discovery of nano-fossils in a meteorite from Mars. Suddenly, as one scientist put it, NASA was bitten by the life-on-Mars bug. The discovery, by a team of NASA scientists, gave the agency a focus it had been lacking since the Challenger disaster a decade earlier. The following year, the Pathfinder spacecraft settled on Mars on the Fourth of July, and its miniature rover rolled down a ramp and inched across the surface of the Red Planet, acting as a robotic geologist. “We can now get to the Red Planet for the price of a big-budget Hollywood movie,” NASA claimed. Jim puts it even more simply: “Mars is back.” It’s his mantra.
The Keflavik Naval Air Station, where Jim and I are billeted in Iceland, is a sprawling NATO base that once served as an essential Cold War outpost. These days, it’s mostly a stopover for young European pilots who bring their planes in from France or Italy; they drink a lot, sleep a little, and depart at first light. Although Jim is a civil servant, his quasi-military status becomes evident the moment he enters the base. He salutes everybody, and they salute back – at least, some do. “My civil service status grade is equivalent to a Colonel’s,” he says, “but no one here is aware of that.”
We’re assigned to the Bachelor Officers Quarters, cement barracks strongly reminiscent of college dormitories. The penetrating odor of burned pizza crust wafts through the halls; the walls reverberate with blasts of heavy metal music. Occasionally, you hear squeals and shouts from girls who may or may not belong here. When you look out the window, you see a landscape so flat and featureless it could be Nebraska. There are schools, playgrounds, pickup trucks, a movie theater, a bowling alley, and a Wendy’s where they play “God Bless America,” country-style, over the PA system. The unofficial motto of the base might be: “Keflavik, a Nice Place to Raise a Family.”
In July, it’s light all the time, and the only way you can tell it’s late in Keflavik is that it gets very quiet. For a few hours, there are no cars zipping around the roads, no fighter jets streaking overhead. Around midnight, there’s a sort of dusk, a suggestion of darkness like a shadow across the sky, but it soon passes, and brightness returns by 2 AM or so.
A few days after our Surtsey expedition, Jim goes forth in search of glaciers to measure. We head out in a Land Rover Discovery across the treeless, craggy, doom-laden landscape, in which people, or, for that matter, all life forms, even grass, seem out of place. Mars on Earth. “You have to remember, Iceland, except in the highlands, looks like the ocean floor,” Jim says. “Now, what if I were Spock in ‘Star Trek,’ looking at the Earth from the Starship Enterprise? Captain Kirk says, ‘Spock, what do you see? Put the scanners on.’ I’d say, ‘I see a watery planet. It’s a planet dominated by oceans.’ The land is an insignificant fraction of what makes up this planet. If we could peel away the water and look at the Earth from space, planetary scientists would say, ‘I see what the Earth does. It has a large system of very thin crustal blocks that are moving and being eaten up in some places and being regenerated in others.’”
Jim catches his breath and swerves to avoid a small herd of scrawny Icelandic sheep. “Now we are starting to add a tapestry of new measurements from Mars Global Surveyor, as we try to understand all these different surface units on Mars. Scientists want to find hot pits, if there are any, just like the ones you saw on Surtsey. Now, how big were they on Surtsey?”
Just a few inches wide, I remind him, and he points out that it would be very difficult to see such tiny formations from space, even at high resolution. “You would need an extremely sensitive thermal scanner in orbit.” Such a device actually exists, but it would not, on its own, be able to detect alien life. Scientists also look for biomarkers, that is, distinctive signatures of life. And they seek signs of an energy or nutrient system capable of sustaining life. “On Mars, we want to find playas, dried up sea-beds, where there might have been standing bodies of water. We see playas on Earth, in the dried lake beds of the western United States, the dry lakes of Australia. On Mars, these playas may be even bigger. The topography measured by the laser going around Mars can find those areas for us.” So playas may hold clues to life on Mars, and volcanoes may also lead scientists to Martian gardens of Eden. It may just be Garvin’s bias, because he is crater expert, but he thinks volcanoes are an important component in the design for living – another reason that Iceland appeals to him. “Iceland has volcanoes that are active, with ice, certainly something that happened on Mars. We have volcanoes interacting with ground water, very important, because there may be ground water on Mars. We don’t know. And we have volcanoes here producing new lava at great rates. Some of the volcanoes on Mars have sustained high eruption rates for hundreds or even thousands of years. That’s what it takes to make an Olympus Mons” – and Olympus Mons is so big that it couldn’t exist on Earth. “There’s too much gravity here, and anything aspiring to Olympus Mons-like grandeur would collapse under its own weight.” He likens its shape to the much smaller lava shield volcanoes of Iceland. The term is meant to suggest a Viking shield turned on its side; a lava shield volcano slopes very gently. “It’s the most common landform made by volcanism in the Solar System. Mother Nature does not know how to do it any simpler.”
Later, we coast past an immense, dry lake bed studded with pebbles. We get out and walk across its dusty surface. It would not be surprising to see a pterodactyl soar overhead, or a spacecraft descend from the skies. This is Nature’s rough draft, a land of possibilities. It’s not as polished as later versions, but the crude landscape yields its secrets and intentions to geologists. “When the water dries up, it leaves behind a lag deposit of rocks,” Jim remarks. The rocks range in size from small cobbles up to large boulders. “And anything bigger,” he announces, “is called a real big boulder! The bright stuff you see here is a layer of desiccated, cemented dust made of clay. That is what comes out of suspension when water evaporates. We expect to see signatures of that kind of stuff on Mars.” He points to a fissure in the soil. “See this desiccation crack? This is what we hope to see on Mars.”
We head north until we reach an enormous glacier: Langjökull. On the other side, its summit obscured by cloud cover, is the great volcano known as Ok. The stony, dusty ground, reddish brown, contrasts with the huge wall of ice. I slowly become aware of the landscape’s resemblance to images of the Martian icecaps, those vast dull white fields rising out of the reddish Martian desert. The more we look, the more striking the resemblance to the northern latitudes of Mars. Our isolation feels complete. No birds or cars disturb the pure silence. No airplanes streak by overhead; the atmosphere is untarnished by plumes of smoke. The spectral glacier rises impressively from the dark red rock, its façade reaching into the clouds and mist, massive, gloomy, impersonal, hypnotic. Nearly everything looks alien and supremely indifferent to the two tiny human figures in the midst of this vast, primeval sanctuary. Take all the measurements you want of Mars, but walking through this strange and unnerving place suggests, as nothing else can, what it would be like to traverse the surface of the Red Planet.
Suddenly a pair of bicyclists disturbs us, a man and a woman, en route to a distant town or campsite. It’s a relief to share the oppressively majestic Martian landscape with others, even briefly. And then they’re gone, gliding into the distance on their bicycles, and we’re alone again. For once, Jim is speechless. We return to the car in silence.
When we reach Keflavik, Jim drives us over to the tarmac, saluting smartly whenever he passes a military guard. At last, the afflicted P-3 aircraft is here. It looks all right from a distance, but a closer inspection reveals oil leaking from the nose, creating an embarrassing, 125-foot long stain on the ground, beginning directly beneath the aircraft. There’s talk that the Keflavik Naval Air Station may insist the P-3 leave immediately so that it does not foul the runway.
Jim trots to the base’s weather station, where the latest satellite data are available. The weather station glitters with state-of-the art equipment; the place is so big and solid it looks like the bridge on an aircraft carrier. Although it’s sunny here in Keflavik today, the instruments reveal there is a weather front moving in, and steel gray clouds are visible on the horizon. It’s now about 3:30 in the afternoon, and sometime after 5:00, the plane is supposed to be in the air, on a six-hour mapping mission. Right away Jim sees it will likely be too cloudy to take data over Surtsey, so instead they’ll survey a floodplain known as the Sandur, located in the Eastern portion of Iceland. Given the weather and mechanical constraints, this will likely be the only day they will be able to take measurements.
Jim sits at a computer terminal in the Weather Station and begins composing a report to the base commander about his activities here in Iceland; at the same time, he chatters with me and an affable young naval attaché. He types rapidly, never making a mistake – “…As part of NASA’s continuing research interests in Iceland as a microcosm for global Earth environmental change and as a natural analogy for landscapes on Mars, an aircraft remote sensing campaign was conducted during the period from 20 July to 26 July, 1998. A NASA P-3 aircraft, outfitted with two scanning airborne laser altimeters, an ice penetrating radar, a nadir-viewing digital video imaging system, and multiple GPS receivers, was deployed to Iceland …” – and when he’s done, he rips his report from the typewriter, drops it off at the base commander’s office, and trots back to the P-3.
He bounds up the ladder to the cabin, which looks like the inside of an Eyewitness News van, crammed with television monitors and wires, strewn with Styrofoam coffee cups, and devoid of creature comforts. Within this funky hi-tech cave, he confers with the navigator, Jon Sonntag, and the two pilots. They plot coordinates. They discuss backup plans. They propose flight paths. “Here’s the game plan,” Jim says, tracing the route on the map with his finger. “Take off, come around … here … and then straight to the Sandur. Surtsey looks really good. Now come over here and do this middle line. That’s the number one priority. If that looks good, see if we can do the north line. At that point, we call the option for doing the south line. If it looks like there are no clouds over this icecap, we might be able to sneak up and come around. In the past we always went way up here and came down. I’m afraid that, unless we can throw a real sharp turn, we can’t do it. We fly at eighteen hundred feet.”
All they need is a working airplane. José’ is the mechanic responsible for maintaining the leaky P-3. He stands about five foot three or maybe four, stocky, with a scruffy, uncertain beard, and a good-natured grin. Jose’, who’s American, likes his wine, and he likes his beer. In the evenings, he’s the first to hit the strip bars of Reykjavik, such as they are. The fate of the P-3 now rests in his hands. Even as they tell me stories of his wild doings, everyone about to fly on this plane expresses confidence in him. (Frankly, I wouldn’t let him near my car.) He works slowly and methodically on the plane, and when he’s done, declares it good to go. The engines roar to life.
The white P-3, with Jim inside, taxis far out onto the tarmac and ascends to the skies over Iceland. While they’re in the air, the pilots do most of the work, for they have to maintain alignment not only with the surface of the planet below but with the Global Positioning System satellite above. This means the plane can’t wander more than six feet off course. They map the Sandur, and, weather predictions to the contrary, they do Surtsey, as well. They do their mapping with reference not to the Earth’s surface, which is always shifting, but to Earth’s center, which is about as close to an absolute, fixed point as you can get.
Mission accomplished, the P-3 returns to Keflavik after midnight. To everyone’s surprise, the aircraft has performed flawlessly that evening, laying down precise tracks over Surtsey and a glacier to the north. “We laid down fourteen lines!” Jim announces. “It was fantastic. Staggered just the way we wanted them. And the weather was great. It was sunny and clear on the island. I’ve got digital video, nose cone video. We’re going to have the best map of Surtsey ever made, no question. The flight was as tranquil as bath water. Even the leak stopped by the time we landed.”
To celebrate, Jim and Jon Sonntag and I go out for a beer. After a long day’s work in the field, no self-respecting NASA scientist thinks of anything but a beer. The drinking etiquette is to avoid brand names and even recognizable microbreweries in favor of obscure local product. Eventually, we find a little place overlooking the large bay, where they serve frosted glass tankards of Viking, an Icelandic beer. We sit in front of a picture window overlooking the steel gray expanse of Keflavik Bay. A few lights flicker across the water, but not many.
Having just spent the last five hours in geological nirvana, Jim talks on and on about what a great mission it’s been, while Jon brings up a slightly different subject: the kind of woman he’d like to meet and settle down with. He’s from Houston, but he wants to meet a different kind of girl from the ones he’s known in Texas. Maybe here, in Iceland. Maybe even in New York City. He asks me about the women in New York, where I live, and I tell him the best thing to do is visit and see for himself. He pauses and smiles shyly, contemplating the prospect. He just might do that.
This type of talk makes Jim uncomfortable. Throughout our time in Iceland, a lot of stray remarks have escaped his lips about power tools, about the power washer he was using just the other day with his son Zack (“That thing was so powerful,” he said with genuine conviction, “that it could take the paint right off a car”), about the Ford F-150 pickup truck he’d like to own, and about a Hummer (“How much do those things cost?”), but nothing about women. Which doesn’t mean that women don’t look at him. They do, indeed. His handsome dark Irish looks, his snappy NASA flight jacket, and his politeness combined with an occasional air of confusion tend to attract women.
He met his wife Cindy by accident in 1990 when she was working at NASA for a contractor. It seems there was another J.B. Garvin with whom she was doing business, and Jim kept getting e-mails intended for the other one. So he got in touch with her to clear up the confusion. He found himself talking on the phone with her, and she coaxed him gently into asking her on a date. “I often get too focused on my work. I wished I didn’t, but that’s the way it is,” he confides. Cindy was determined to change all that.
When they came face to face, Cindy already knew what he looked like; to this day, Jim is not sure how she knew. They went to a hockey game, and not long after that, moved in together. Cindy recognized that his interests were a little unusual. Here’s a Phi Beta Kappa from Brown, a Ph.D., who says his most valuable possession in the world is a complete set of Jim Bunning baseball cards. He owns practically every Bunning card ever issued, tracing his pitching career from 1954 through the 1980s. Cindy liked shopping, dining out, and other normal activities. That was fine with Jim, he wanted to be with someone normal, someone who would keep him in touch with daily life. They married in 1992. We sit drinking and talking until the sky begins to brighten almost to daytime intensity, and we return to base a little after two in the morning.
The next day, while packing to leave, Jim ponders what to do with the data he’s collected during the week in Iceland. He must get it out – in the new NASA, nothing is secret – so he will post it on various Internet sites, for starters. He will write multiple papers, some of which will appear in scientific journals. He will give lectures. He will share the information with Icelandic scientists. “It’s my job as a research scientist at NASA to publish the results in Science, Nature, and other journals. That’s my job, to disseminate.” He will have plenty to discuss, for a crowded schedule of Mars exploration lies ahead. “We’re launching again in December and sending a small probe to the south polar ice cap on Mars, which we think is all frozen carbon dioxide. It’s so cold, one hundred to two hundred and fifty degrees centigrade below zero. We’re also asking other, very fundamental questions: Did life start on Mars? If it did, is it dormant, frozen, fossilized? Is it still there? Is it all microbial? What can it tell us about extremophile life on Earth?” Jim asks, savoring each question.
“I think the potential for Mars is totally untapped, and that’s something of a surprise,” he continues. “When we first got there in the sixties with the Mariner spacecraft, we thought, ‘Oh, my God, there are going to be Martians, canals, it’s going to be great.’ But when we got there, it looked like the moon. Mars puzzled us. We returned with Viking in the mid-seventies, looking for life, and instead we found the great arctic desert of Mars. We saw frost form in the winter, and we saw snow. We saw rocks and pits that reminded us of gas bubbles in the volcanic rocks you see here in Iceland, but we didn’t see the obvious signatures of life. We’ve got to go back. We’ve got to understand this place. We’ll have a series of robotic voyages to set the stage for bringing back samples of Mars to Earth to investigate the chemistry and – maybe – signs of life. And then someday we’ll put human beings there, God and the great American economy willing.”
The taxi cab heading home from JFK Airport feels as cramped as Oscar’s co-pilot seat. I’m probably in more danger barreling along the Grand Central Parkway than I was aloft in Oscar’s little Aerospatiale. Night falls for the first time in a week; how strange the darkness seems. After experiencing Iceland’s white nights and thinking intensely about what it’s like to walk across the surface of Mars, I find that nothing on Earth looks quite the same. The initiation is over, and back home, I reflect on a whimsical passage from Ray Bradbury’s sci-fi novel, The Martian Chronicles, published in 1950:
The ship came down from space. It came from the stars and the black velocities, and the shining movements, and the silent gulfs of space. It was a new ship; it had fire in its body and men in its metal cells, and it moved with a clean silence, fiery and warm. In it were seventeen men, including a captain … Now it was decelerating with metal efficiency in the upper Martian atmospheres. It was still a thing of beauty and strength. It had moved in the midnight waters of space like a pale sea leviathan; it had passed the ancient moon and thrown itself onward into one nothingness following another. The men within had been battered, thrown about, sickened, made well again, each in his turn. One man had died, but now the remaining sixteen, with their eyes clear in their heads and their faces pressed to the thick glass ports, watched Mars swing up under them.
“Mars!” cried Navigator Lustig.
“Good old Mars!” said Samuel Hinkston, archeologist.
According to Bradbury, this landing, the third human expedition to Mars, was supposed to occur in April 2000. NASA is running a little behind schedule, but sends spacecraft to Mars as often as budgets and planetary orbits allow. For now, they are robotic missions; in time, they will bring people to the Red Planet.
Welcome to the new Martian chronicles.
2 MESSAGE IN A BOTTLE (#ulink_dfa6ed39-e414-52ec-8daf-cbd488ac9866)
Jim Garvin’s collection of Jim Bunning baseball cards got me to thinking about what is perhaps the most famous baseball card of all – the 1909 portrait of Honus Wagner in a Pittsburgh Pirates uniform. When I gaze at the face of this young man, who seems to be staring into space, I find myself asking, “What was it like to be alive in 1909?” I have little idea, although it was just the other day, in geological terms. All I have to go on are artifacts, such as this famous baseball card. I can’t watch Wagner play baseball, and I can’t hear his voice; all I can see is a fuzzy image of the athlete in a uniform, a trick of light and shadow, an impression of life as it once was. To fill in the gaps, I would have to look beyond the card, but if I’m relying on the card, and only the card, I have precious little data.
The card, and its limitations, call to mind the tantalizing images of fossilized bacteria in a 3.9 billion year-old meteorite from Mars – images that may be the first scientific evidence of life beyond Earth. Fossilization occurs when minerals replace organic elements in once living things. The morphology remains, although the chemistry is different. Still, scientists can learn a lot from fossils. They can detect the approximate age, which is crucial, and, by studying fossils in their natural setting – in situ – they can extrapolate a great deal about the geological, chemical, and biological circumstances surrounding them. “Fossils are the autographs of time,” wrote the American astronomer Maria Mitchell. For these reasons, fossilized bacteria from Mars – if that’s what they are – have great appeal; they are our best indication of life beyond Earth. Like the antique baseball card, they offer only a very narrow glimpse into the past. 1909, the year of the Honus Wagner card, wasn’t very long ago, but it’s long enough past to seem quite mysterious. How much more difficult it is, then, to construct a scenario for the existence of life on Mars several billion years ago from the evidence contained in a meteorite.
If the fossilized bacteria are genuine artifacts of Martian life, they raise more questions than they answer. If life started on Mars, how did it begin? Is it still there? If not, when did it end, and why? If it’s on Mars, where else in the universe might it be, and what form does it take? Did it originate on Mars, on Earth, or somewhere else? All these Genesis Questions point up how much scientists have yet to learn about how life began. In the course of asking questions of various scientists who study these problems for a living, almost every reply I received began this way: “No one knows, but …” That’s followed closely by, “There are several possible scenarios,” and “Well, current speculation has it that …” The answers are all variations on the theme that no one knows, yet. But scientists have hypotheses. They have scenarios. The meteorite from Mars has inspired a widely accepted scenario – I’ll call it the Best Guess Scenario – concerning the origins of life on the Red Planet, and how it came to be transported to Earth.
Four and a half billion years ago, the Solar System was in its infancy, and the planets were new. In its first billion years of existence, Mars was a warmer and wetter place than it is now. Water flowed freely over its surface and pooled underground, in reservoirs. The flood channels carved into the surface of Mars, some of them many miles in width, left an eloquent record of catastrophic outpourings of water. In all likelihood, the water on Mars was quite salty. (Fresh water on Earth is due to evaporation and rainfall.) Eventually, the floods subsided, and the water drained into Mars’ vast northern plains, where it might have frozen. In the process, it reshaped the Martian terrain until it resembled a desert that had once been flooded, but became bone dry. Nevertheless, its contours preserved geological memories of rivers and oceans and lakes.
The large Martian pools of standing water were subject to peculiar tides caused by the planet’s two small moons, Phobos and Demos. And they were subject to the Martian winds; when they blew, reaching speeds of hundreds of miles an hour, they generated waves with peculiar shapes, higher and steeper, with more pronounced peaks than exist on Earth. The lower Martian gravity, less than half of Earth’s, allowed the slender waves to tower until they resembled the watery shapes in a drawing by Dr. Seuss; they would flop over and spatter, as if in slow motion. The marine scene on Mars was all oddly familiar, and strangely different.
The Martian sky was blue a few billion years ago, and there were a few clouds, just as Mars has now. It was mostly cold, and extremely cold at the poles, except for the equator, where it was warm. Martian volcanoes erupted with regularity, and in the Red Planet’s low gravity they assumed formations that couldn’t exist on Earth; they were larger and higher. In these ancient Martian conditions of two or three billions years ago, life could have formed and evolved, just as life appeared on Earth within a billion years of this planet’s existence. The volcanoes, especially the ones close to reservoirs of water, or polar ice, created hot spots where life would most likely have formed on Mars. No one knows how far it developed, or if it ever got underway. It might have remained dormant most of the time, for tens of millions of years at a stretch. Or it might have progressed beyond simple bacteria; there might have been Martian insects crawling around, adapted to the Red Planet’s lower gravity, lower density atmosphere, and cooler temperatures. These variations suggested life forms that were spindly, similar to insects. The skeletons might have been external, with many legs to take advantage of the lower gravity. As for the cooler temperatures, life on Earth has shown remarkable adaptive creativity. “Some insects winter-proof themselves with glycerol, a common antifreeze used in automobile radiators,” Carl Sagan theorized. “There is no conclusive reason why Martian organisms should not extend this principle, adding so much antifreeze to their tissues that they can live and reproduce in the extremely cold temperatures occurring on Mars.” The ancient Martian atmosphere would have required similar creativity in the creatures’ breathing apparatus. If they had evolved to the point of multi-cellular differentiation, they might have developed enormous gills or lungs, relative to their size. Even if life never reached this advanced stage of evolution on Mars, it is still possible that tiny organisms formed in the water-drenched Martian rock, and then, for some reason, died off, leaving fossilized remains hidden beneath the surface. It was as though Nature initiated an experiment but abandoned it in the early stages.
Ancient Mars was more turbulent than Mars is now. In the young and volatile solar system, it was constantly bombarded by chunks of asteroids. It is possible that at some point in Martian history, an asteroid of such dimension struck Mars and created cataclysmic changes in the planet’s climate and geography that whatever life forms had managed to take hold were snuffed out, leaving only their skeletons, which became fossilized. Or perhaps the death of Martian organisms came about slowly, as the planet lost its atmosphere a little at a time to space, and its water eventually disappeared below the surface, or vanished with the atmosphere, leaving behind a desiccated, celestial sandbox.
If we had been able to observe the first few billion years in the life Earth and Mars from a vantage point in distant outer space, we might have noticed several common trends. We would have seen watery places on both planets. We would have seen volcanoes on both planets, their plumes of smoke, their pollution of the atmosphere. We would have seen clouds on both planets, and we would have detected seasonal waxing and waning of the polar caps. As the eons passed, subtle differences between the two planets would have become apparent. If we had been looking closely, we might have noticed the atmospheric changes. We might have seen the dramatic increase in oxygen in Earth’s atmosphere, and a corresponding spread of vegetation on its surface; if we were very perceptive, we might have noticed the spread of plant life in its oceans, in the form of algae.
At roughly the same time, we would have seen that Mars was losing its nitrogen-rich atmosphere. It was thinning out, disappearing into the frigid vacuum of space. More obviously, we would have seen the great Martian standing bodies of water recede, exposing a complex erosional system of gullies and playas and rearranged boulders, many of them acting as signposts to the water’s former whereabouts and actions. During the last few hundred million years, if we were sufficiently attentive, we might have noticed the spread and intensification of vegetation on Earth, as the biomass increased and diversified, and various life forms competed for natural resources, or evolved ways to cooperate, or both. At about the same time, we might have watched Mars continue to regress to its early state, with some important differences. It contained geological traces of water, and perhaps traces of biology – clues, ultimately, to its origins, and to ours.
Where did the elusive Martian water and its life-giving properties go? Come to think of it, where did the water come from? Where did water on Earth come from, for that matter? At the Lunar and Planetary Institute in Houston, Steve Clifford has spent years studying water on Mars, and he told me there are several schools of thought concerning the origins of water on this planet and on Mars. “One is that after the Earth was formed, comets bombarded the planet, adding volatiles over perhaps the first billion or half billion years.” A comet is basically a celestial snowball, bearing ice from somewhere – God knows where – to here. “The other school of thought is that much of the water we have on the Earth was contained in the early material that formed the planet. As the Earth started to accrete asteroidal material and dust in the early Solar nebula, it gradually reached a size where the quantity of radioactive material was sufficient to heat up the planet and cause it to differentiate. The heavier stuff sank toward the middle, which is how we got an iron core, while the lighter stuff, which may have contained water, was released during the formation of the crust and atmosphere.” A similar process may have occurred on ancient Mars at about the same time it happened on Earth.
Steve surprised me by suggesting that water on Mars may still linger beneath the surface, more than a little. He was talking about “sizable reservoirs of ground ice and ground water.” The evidence he has for large volumes of water on Mars is mostly indirect. He calculates the amount of “pore space” to be found in Martian rocks and soil; water could be stored there. If it is, some of the water could be in liquid form, especially well below the surface. “Like the Earth, Mars is thought to be radiating internal heat due to the decay of radioactive elements, which means the deeper you go below the surface, the warmer the temperature gets,” he says. “And if you go down several kilometers, you could easily get temperatures that are consistently above freezing,” which means liquid water might exist on Mars today. In fact, he thinks there might be two types of water reservoirs on Mars, a region of permafrost near the surface, as well as larger and warmer reservoirs at greater depths. There you might find liquid water in great quantities, and water, of course, leads to life. This subsurface system would act as a powerful preservationist of life, no matter how harsh conditions on the surface. “If life ever evolved on Mars, and adapted to a subterranean existence, then its survival would be assured for the indefinite future.”
Subterranean life on Mars could survive the loss of the atmosphere, it could survive intense cold on the surface, it could even survive the largest life extinguisher we know about, the impact of a large asteroid. Imagine what would happen if an asteroid the size of Manhattan collided with Earth, Steve says. It would certainly be calamitous and likely sterilize the surface of the planet down to a depth of several meters or so, but not below that. It would kill life on the surface, but it wouldn’t eliminate all life on Earth, because the impact’s thermal and chemical effects would be limited to the uppermost part of the surface, with the exception of the area where the impact occurred. After the impact, life far below the surface of the Earth would go on. The same holds true on Mars, Steve says: “any microorganisms that might have evolved four billion years ago, when the planet might have been warmer and wetter at the surface, could readily survive to the present day at depths of several kilometers.” If he’s correct, or even partly correct, life could very well exist within the Red Planet’s ancient reservoirs, awaiting discovery.
The Best Guess Scenario assumes that some kind of simple life did exist on Mars a few billion years ago, as Steve described. And it assumes that asteroids have bombarded Mars ever since, pockmarking its surface with craters. Sixteen million years ago, according to the scenario, a refugee from a disorganized asteroid belt struck the surface of Mars with tremendous force. Since Mars has only 38 per cent of Earth’s gravity, the impact was sufficient to drive pieces of rock buried beneath the surface high into the Martian sky and beyond. The pieces shot into space five times faster than a bullet – fast enough to escape the Red Planet’s gravity. Until a few years ago, it was thought the shock of impact would vaporize or severely deform the ejected material – the ejecta – along with everything in it, including any signs of life, but recent computer modeling has shown that the physics involved would allow the ejecta to remain intact. In the model, the asteroid comes in at an angle, strikes, and creates a vapor cloud that sweeps across the surface at an extremely high speed and carries material off Mars into solar orbit. The whole process might take five or ten seconds, long enough for some fragments of Mars to remain intact. “That’s a fairly gentle way to get stuff off the surface,” says Mark Cintala, who studies craters at NASA’s Johnson Space Center – gentle enough to launch a fossil-bearing meteor on a trajectory to Earth.
There’s a variation in the Best Guess Scenario that incorporates another method of ejecting material safely from the surface of Mars: spallation. Much of the work on the spallation model was done by Jay Melosh at the University of Arizona, and it’s extremely simple, in theory. You put a quarter on a tabletop, rap the underside of the table, and bump the quarter into the air; that’s spallation. “You’re sending a stress wave through the table, and that stress wave is transmitted to the quarter. Imagine that happening on Mars,” Mark Cintala says. In that case, the asteroid’s impact would create a compressional wave, as if it were a depth charge below the surface. The resulting shock wave would bounce a rock fast enough to escape the relatively weak Martian gravity and send it on its way. “At least, the calculations say it can.”
In the Best Guess Scenario, one of these dislodged pieces of Martian rock sped off in the direction of Earth, a cosmic message in a bottle floating through an ocean of outer space. The journey lasted millions of years, and the ancient chunk of Mars crashed to the surface of Earth a mere 13,000 years ago. The size and shape of a potato, it buried itself in the Allan Hills of Antarctica. The meteor had become a meteorite.
Meteorites have held a special fascination as relics from the heavens, mute messengers from parts unknown. In the Middle Ages, meteors falling to Earth generated superstition and concern. Where did they come from? What did they mean? The faithful brought them to the authorities, and in time, the Catholic Church acquired a large repository of these curiosities. In 1969, the study of meteorites underwent a quiet revolution when Japanese researchers found high concentrations of them preserved in arctic ice. Since 1977, NASA, a technological Vatican, has been collecting meteorites from Antarctica and housing them at the Johnson Space Center in Houston. Each year, there are hundreds of new arrivals, and when there’s a promising delivery, scientists clamor to get a piece to study. There are now nearly 10,000 rocks under lock and key in Building 31 at Johnson, many of them preserved in nitrogen. By measuring the radiation absorbed by the meteor during its space travels, scientists can determine approximately when the rock arrived on the Earth, and even how long it spent in space before it arrived on our planet.
In December 1984, Roberta Score was hunting for meteorites in Antarctica. At the time, she was employed by Lockheed Martin and working at the Johnson Space Center. Around Johnson, a meteorite collecting mission is not exactly choice duty; join one, and you were said to have become part of the “Houston weight loss program.” Walking across an apparently endless sheet of ice, Robbie Score came across a greenish stone about the size of a potato. Once she removed her sunglasses, she saw the meteorite was not greenish, after all; it was gray and brown, but she knew it looked different from the ordinary meteorites she found in the field. Along with other samples, it was kept in a freezer aboard the ship that brought it from Antarctica to Point Magu, California, where it was packed in dry ice and sent to Johnson, where it was stored in cabinets that once held moon rocks. The meteor curators, including Robbie Score, designated it ALH 84001 – their way of saying this was the most interesting meteorite collected in 1984 in the Allan Hills of Antarctica. But after being delivered to Johnson, ALH 84001 was misidentified as an asteroid fragment, a diogenite, rather than a piece of Mars, and stored in Building 31. It was not ignored, however; small sections were allocated to the scientific community for further study over the years; in all, almost a hundred “investigators” examined it, and everyone continued to misclassify it as a diogenite – with one exception.
In late 1993, David Mittlefehldt, a veteran Lockheed Martin scientist also working at Johnson, reexamined ALH 84001. Mittlefehldt was an expert on diogenites, and this particular rock didn’t look like one to him. It seemed to have more oxidized minerals in it than your normal diogenite, for one thing. Using new technology in the form of high-resolution laser spectrometry, two other scientists, Donald Bogard and Pratt Johnson, extracted gases trapped inside the strange meteorite and discovered that their very idiosyncratic characteristics exactly matched gases on Mars as measured by the Viking spacecraft in 1976. Mittlefehldt published his findings in 1994 in a scientific journal, and attracted the notice of the science community. Although this wasn’t the first meteorite from Mars to have been discovered, the reclassification created a stir. Of the thousands of meteorites that have been cataloged, only fourteen are believed to have come from Mars; the overwhelming majority come from asteroids, and a few from the moon. Meteorites are named for the places where they have fallen to Earth, so the Martian meteorites have some fairly exotic names – Shergotty (India), Nakhla (Egypt), and Chassigny (France), among them – and are known collectively as SNC or “snick” meteorites. “SNC meteorites” is an elaborate way of saying “meteorites from Mars.”
Carefully considering his find, Mittlefehldt noticed minuscule reddish-brownish areas deep within ALH 84001; they looked a lot like carbonates, and on Earth, carbonates such as limestone tend to form close to water. What made this all so curious was that no one had detected carbonates – and their suggestion of water – in the other Martian meteorites. They were billions of years newer; they probably came from a more recent era in the geologic history of Mars, after the water that once flowed freely across its surface had disappeared. This one, however, apparently harkened back to that warm and wet golden age on Mars. Dating confirmed that the meteorite was indeed very old: 4.5 billion years old, much older than other known Martian meteorites, and it contained carbonates that were 3.9 billion years old. Mittlefehldt wanted to get some idea of the temperature range in which the carbonates had formed billions of years ago, so he went to yet another NASA scientist, Everett Gibson, who examined the very curious meteorite with Chris Romanek; they published a paper in the December issue of Nature in which they said the carbonates had formed at temperatures below 100° C, in other words, at moderate, Earthlike temperatures – “well in the range for life processes to operate,” as Gibson puts it.
By now a line of reasoning was beginning to take shape. The team had their meteorite; it was from Mars. Almost no one disputed that singular fact. And it was very old, when water was thought to exist on the Red Planet. And it had carbonates, suggestive of water, formed at moderate, Earthlike temperatures. With each new discovery, the stakes became exponentially higher. ALH 84001 had gone from being a curiosity to an interesting and instructive case study to a potential harbinger of a scientific revolution. Each new link had been more difficult to fashion than those that had preceded it, and the final link – to life on Mars – would be the most difficult of all to fashion.
Other scientists soon began angling for a piece of the curious, potato-shaped Martian meteorite, among them David McKay. Over the years at Johnson, he’d become known as a solid and reliable scientist, not the type to go out on a limb. Carl Sagan he was not. If you asked around about McKay, you often heard words like “cautious” and “self-effacing,” yet he had a distinct air of authority; he’d published hundreds of scientific papers, and he knew his way around Johnson and around NASA. Over the decades, he’d learned about science and about maneuvering in the world of scientists. He knew about the pitfalls, how quick others were to leap on “discoveries” and tear them to bits. Yet with all his experience, he seemed destined to retire in honorable obscurity, until ALH 84001 came to his attention.
“I’m going to get a piece of that meteorite and look for signs of life in it,” he told his wife.
“Sure you are,” she said.
McKay had a vast storehouse of information and impressions about rocks on which to draw. In his long career, he had looked at perhaps 50,000 of them, and he spent many hours studying the most intriguing he’d ever seen, ALH 84001, with a scanning electron microscope capable of magnifying objects 30,000 times. With this instrument, McKay identified a bunch of – well, they looked a little like miniature subterranean carrots, or worms, or tubes. Whatever they were, they didn’t look like something you’d expect to find in a meteorite.
Again, he turned to another scientist for assistance. Kathie Thomas-Keprta was a biologist who had spent almost a decade studying extraterrestrial particles – space dust – before she focused her attention on the meteorite from Mars. She was accustomed to making do with very little. A specially modified B-57, flying at high altitude for an hour, might collect just one extraterrestrial particle from an asteroid, a particle too small to see but big enough for her to examine under a powerful microscope. When McKay invited her to study a Martian meteorite, she was delighted to have something as big as one millimeter by one millimeter to work on after all those years of studying specks. Even better, she was an expert with a new type of electron microscope that could reveal the mineral composition of the carbonates locked in the meteorite. McKay and Gibson showed her the photos taken by the scanning electron microscope, and they proposed that she examine those peculiar, worm-like structures to see if they were fossils. She listened respectfully to their proposal, and when she got home that night, Kathie told her husband, “These guys are nuts!”
A team of researchers based at Stanford subjected chips of the meteorite to further laser tests, which yielded polycylic aromatic hydrocarbons – PAHs, for short – which are often associated with life. That finding raised more questions than it answered, for PAHs are also associated with inorganic material such as pollution and exhaust. If that were the case, the carbon in the meteorite could be the result of very recent contamination on Earth, not evidence of ancient life on Mars. Additional tests showed that the PAHs were buried inside the meteorite and probably quite old, lessening the likelihood that they were the result of exhaust. It looked like the PAHs came from Mars, after all.
The team felt confident enough to announce some initial findings at the 1995 Lunar and Planetary Science Conference, held at the Johnson Space Center. In the planetary science community, the LPSC is a very big deal, a sort of scientific Super Bowl. If you don’t show up for this event, scientists say, everyone assumes you’ve died, and when you do show up, you come to make news, if you can. On behalf of the meteorite team, Kathie Thomas-Keprta presented a paper about the unusual and provocative features of ALH 84001 observed by the team. The paper stopped short of declaring they had found evidence of life on Mars, even very ancient, very tiny life. In fact, she adamantly denied it to a reporter from the Houston Chronicle who suspected she was hinting at it.
She knew other scientists would soon challenge her findings, no matter how cautiously expressed. Faulty science or clumsy handling of the situation could mar several carefully-tended careers. So McKay and his colleagues ran still more tests on the meteorite with an even more powerful scanning electron microscope designed to inspect rockets for minuscule fissures; this instrument was capable of magnifying objects up to 150,000 times. McKay put a four billion year-old piece of Mars under the microscope, and on his monitor there appeared a bunch of worm-like forms. He printed an image, and gave it to his teenage daughter.
“What does it look like to you?” she asked her father.
“Bacteria,” he answered.
Kathie Thomas-Keprta eventually decided the guys on her team weren’t nuts, after all. Her conversion occurred in Building 31 at the Johnson Space Center one night when she was working late. As she examined the shapes of the nano-fossils in the meteorite, she knew from experience they were of biological origin. “It was gregite, an iron sulfite present in the carbonate. It had a certain morphology known to be produced by bacteria. It was actually a biomarker, a thumbprint left by biological activity. I thought, ‘That’s it. There’s life on Mars.’
“I walked out the door to the parking lot, half-expecting to see flags waving and bands playing, but there was nothing at all out there, just a dark, empty parking lot at night.”
The chain of reasoning was more or less complete. The meteorite was old enough to contain of a record of Mars’ early days, when water was plentiful. It had carbonaceous material; it probably had Martian rather than terrestrial PAHs; and it had gregite, a universally accepted sign of biological activity. Although each distinct link could not be taken as proof, they all added up to a fairly strong argument for ancient life on Mars.
The team, now grown to nine, approached Science magazine. They realized that getting the prestigious journal to accept their paper would be difficult and delicate; they might have to withstand as many as four or five anonymous critiques of their work. Science was tempted by the paper but reluctant to support invalid conclusions, so the publication sent out the manuscript to nine readers. The resulting article relied solely on sober observations and rigorous science, and its title reeked of compromise: “Search for Past Life on Mars: Possible Relic of Biogenic Activity in Martian Meteorite ALH 84001.” The most important sentence was the summary: “Although there are alternative explanations for each of these phenomena when taken individually, when they are considered collectively … we conclude that they are evidence of primitive life on Mars.” In other words, the meteorite offered the first scientific evidence that ours is not the only planet in the Solar System where life emerged. Publication of the issue of Science containing the article was set for August 16, 1996.
When Jim Garvin heard about the impending Science paper, he felt the skin on the back of his neck prickle. “I was dumbstruck,” he said. In 1990, he had looked at another meteorite, Shergotty. At the time, no one realized that particular rock had come from Mars. He borrowed a piece of it from the Smithsonian, where it is stored. “I took it up to our lab and made the measurements I’d wanted to make for impact metamorphism” – looking for evidence of shock waves, that is. “This was a passive measurement, by the way, like bouncing a laser pointer off a rock; we weren’t destroying it.” There he was, examining a piece of Mars without realizing it.
The force of the new paradigm – that life on other planets was probably tiny – spun Jim’s thinking in a new direction. “We were still a few months before the launch of Pathfinder and Mars Global Surveyor, and the question was asked, ‘What could be done with these ready-to-go spacecraft to look for more signs of life?’ ” Suddenly, Jim’s Mars mission had a new reason for being. He had always believed it presumptuous to assume that life existed only on Earth, and he was sympathetic to the meteorite team’s conclusions about ALH 84001. Their research science was rigorous, it was cautious, and it was consistent with the latest findings concerning extremophile life. There was something in that meteorite that could not be explained away by conventional arguments. Jim agreed with the team that the burden of proof had now shifted to those who insisted there was no life on Mars. If that was the case, he said, “an interesting explanation as to why life failed to make at least a tenuous foothold would have to be crafted.”
The midsummer Martian madness started in earnest a couple of weeks before publication of the article, when Dan Goldin, the mercurial, publicity-loving head of NASA, heard that Science had accepted the article for publication. Next, the White House wanted to make a grand occasion out of the discovery of possible life on Mars. In preparation for the announcement, Goldin summoned David McKay and Everett Gibson to Washington. “We had thirty minutes scheduled with Dan to talk about the meteorite,” Everett told me. “After an hour and a half, Dan said, ‘You guys take a break, I’ve got some things to do, and then we’ll continue.’ During the break, he dictated a commencement address he was going to deliver at UCLA and handled a few other things, and then we continued for another hour and a half. I felt like I was giving an oral defense of a Ph.D. thesis. I mean, Dan went back to first principles, and he took twenty-eight pages of notes.” At the end of the ordeal, Dan Goldin had one last question for the two scientists: “Can I give you a hug?” The gesture was pure Goldin. In general, NASA is not a touchy-feely place – but Goldin is a man of enthusiasms.
After that, the story began to leak everywhere. Space News, a weekly trade journal, hinted at the forthcoming Science paper about ALH 84001, and the buzz preceding an important Washington story started; then things suddenly went awry. At the stylish Jefferson Hotel in Washington, Dick Morris, an advisor to President Clinton, told a prostitute named Sherry Rowlands about the discovery, in the vain hope of impressing her. “Is it a bean?” she asked. Well, no, not really, he replied. It was, uh, more like … a “vegetable in a rock.” When Rowlands got home and opened her diary to write about her day with Dick, she noted, “He said they found proof of life on Pluto.” Scientists dread being misunderstood by the public, but who could have imagined the magnitude of misunderstanding generated by this discovery? The situation deteriorated even further when the befuddled hooker tried to peddle the story to the tabloids, which turned out to be more interested in extraterrestrial life in the form of little green men than vegetables in rocks, thank you. And her inability to recall just what planet Dick said they’d found life on – Saturn, maybe? – didn’t help her credibility, either. There was no sale.
The life-on-Mars story quickly took on a life of its own. The CBS Evening News was making disturbing noises that it might break the news even before confirmation, according to an account that appeared in Texas Monthly. Other networks sensed news in the making and assigned reporters. Science tried to halt misunderstandings by posting the article on the Internet shortly before publication. On the first day alone, the website received over a million hits. Giving substance to an age-old dream, and terror, the article’s findings excited worldwide attention. The announcement gave new impetus to America’s expensive, beleaguered space program, especially its investigations of Mars. Goldin was delighted to confront a challenge of this magnitude, and the mood surrounding it recalled the great days of the space race, when Americans had an emotional investment in NASA and the nation’s fortunes seemed to rise and fall with the agency. But the issue of life on Mars was more complicated to explain to the public and sell to Congress than sending people to the moon had been. There was no life-on-Mars race for politicians to exploit. National security and national pride were not at stake. Only the science really mattered. The discovery involved concepts difficult for most people, even scientists, to understand, including a meteor of unimaginable age that had traveled to Earth from an unimaginable distance, containing evidence of life that was unimaginably tiny.
NASA finally made the announcement at a flashy press conference, at which an exuberant Dan Goldin proclaimed, “What a time to be alive!” (And the head of NASA, he might have added.) Bill Clinton, campaigning for reelection, appeared on the South Lawn of the White House to hail the discovery as if it were another triumph for his administration, but he actually sounded a note of caution that went largely ignored: “If this discovery is confirmed, it will surely be one of the most stunning insights into our universe that science has ever uncovered.” That was still a big if. And his declaration that the American space program would now “put its full intellectual power and technological prowess behind the search for further evidence of life on Mars” did not necessarily mean additional money for a beleaguered NASA. His words amounted to a mere presidential pat on the back.
The summer of Mars was underway. For a while, the names of the several NASA scientists on the meteorite team – McKay, in particular – were known to journalists and the general public. The sudden popularity threw the scientists for a loop. They naturally desired professional recognition, but not celebrity. In their line of work, being famous meant being considered suspect, a semi-charlatan, a talking head rather than a working research scientist. None of them aspired to become the next Carl Sagan, bridging the gaps among the media, the scientific community, and the public. Although their thinking was revolutionary, they weren’t visionaries; they just wanted their funding, and they wanted to pursue their scientific interests. The announcement concerning ALH 84001 made it harder for them do that, as publicity insinuated itself into the normally orderly process of disseminating scientific information. Instead of addressing specialists at conferences and publishing in specialized journals, science teams proclaimed their findings in press releases, in advance of publication. Freed of the constraints imposed in a refereed publication such as Science, the releases tended to make larger claims than the articles that inspired them. Conducting science by press release troubled many, including those engaged in the practice.
The announcement concerning ALH 84001 transformed NASA. For the first time, many people realized that NASA supports scientists, not just astronauts and engineers and the crews that send them into space. In its youth, NASA had accomplished one spectacular engineering feat after another: putting an astronaut in orbit, sending astronauts to the moon, keeping astronauts in orbit for months on end. These missions included science, but science was rarely the point. Flags and footprints on the moon were the point. Astronauts did collect a few hundred pounds of moon rocks for scientists to analyze, but the public had scant interest in lunar geology. Now, with the announcement of possible nanofossils in ALH 84001, NASA scientists were no longer overlooked. And with the end of the cold war, they could participate in missions that were primarily scientific rather than political, missions that might become more significant than sending people to the moon. They suddenly had an opportunity to devise experiments exploring fundamental questions about the nature of the universe and the origins of life. Their results of their search, a NASA report concluded, “may become a turning point in the history of civilization.”
The message in a bottle had arrived, but who would decipher it correctly?
Throughout the summer of 1996, David McKay expected a backlash concerning his discovery, but it was slow in coming. At first, members of the public, some of them deeply suspicious of all federal agencies, NASA included, sent him angry e-mails, most of which echoed the theme, “What kind of fools do you take us for?” One said, “Your life on Mars story is a good example of your mistaken belief that the general public is comprised of a bunch of total idiots.”
Eventually, scientists joined the clamor. Some insisted that ALH 84001 proved absolutely nothing. The wormlike structures, said critics, were far too small to be bacteria; in fact, they were many times smaller than the smallest bacteria ever seen on Earth. Others insisted that if the meteorite contained evidence of biological activity, it was the result of contamination. Still others challenged the team’s analysis of the PAHs. Some scientists stated flatly that McKay and his team had unfairly manipulated the evidence to support a flawed hypothesis. Everett Shock at Washington University invoked the Murchison meteorite, believed to have come from the asteroid belt, to invalidate the discovery. “It has carbonate minerals in it,” he said, “and real solid evidence of water – yet there isn’t anybody saying that there is life in the asteroid belt.” True, no one was saying it at the time, but that situation is beginning to change as scientists have come to think of life as widely distributed throughout the Solar System. Finally, the scientists attacked the reputations of McKay and his team, a tactic that took cooler heads by surprise. “It’s kind of strange when scientists, who are thought to be rational, become emotional,” said Marilyn Lindstrom, a curator of meteorites at the Johnson Space Center. “What bothers me most is that so many people have made up their minds before the data come in. I mean, sometimes I’m amazed by McKay and Gibson’s almost true-believer attitude.”
Carl Sagan was seriously ill at the time of the announcement, with only a few months to live. During his decades with NASA, he had become familiar with both the science and the passions involved in the search for life beyond Earth, and his pronouncement on the subject was enlightening yet equivocal. “For years I’ve been stressing with regard to UFOs that extraordinary claims require extraordinary evidence. The evidence for life on Mars is not yet extraordinary enough. But it’s a start.” Although he was deeply intrigued by the meteorite team’s findings, Sagan insisted that more study was required. Yet other scientists were convinced by McKay’s rigorous approach. “If this is not biology,” said Joseph Kirschvink of Caltech, “I am at a loss to explain what the hell is going on. I don’t know of anything else that can make crystals like that.”
Because McKay, Gibson, and company were cautious, even cunning, in the way they stated their findings, they made it difficult for their critics to disprove their argument. The meteorite team held that the fossils were merely possible evidence of relic life; they were not the only explanation for what they’d found, merely the best explanation. To disprove or dismiss these findings, their critics would have to understand ALH 84001 even better than the original investigators did. They would have to refute four separate, interrelated lines of argument. They would have to be familiar with geochemistry and physics and geology and of course biology. No one person knew enough about all these fields as they applied to the meteorite; it would take a team, a bigger and better team, to show McKay and his colleagues the error of their ways.
The significance of the debate transcended the meteorite itself. Even if it contained crystals that mimicked biological morphology, or contamination, the search for extraterrestrial life had undergone a sea change. Even scientists who thought ALH 84001 contained no life signs at all now found themselves thinking that if we were going to find evidence of extraterrestrial life, it would probably be tiny and ancient and carried throughout the Solar System in a meteorite. McKay, Gibson, and Thomas-Keprta’s real discovery was a new paradigm. Even if their conclusions turned out to be incorrect, their thinking was too sophisticated to dismiss. From now on, they would define the terms in the search for extraterrestrial life. Their credibility rested not so much on what they found as on how they found it: their precise, rigorous methodology.
Two years after the announcement, I found Kathie Thomas-Keprta in the featureless Building 31 at the Johnson Space Center, where many of the crucial discoveries concerning the meteorite had occurred. She is tall and slender, with long blond hair swept up in back. Despite the intense debate concerning her work, she didn’t look embattled; she was poised, with a certain swagger and the smooth delivery of a television talk show host, at least in one-on-one conversation. We were standing beside another Martian meteorite, EETA 79001, a cousin of the more famous ALH 84001. EETA 79001 resembles a black ice cube, about two inches by two inches. I peered carefully at this Martian specimen. There wasn’t much to see except for a little hole in one side drilled by a laser to extract gases trapped within.
Her team expected a lot of debate after their discovery, she told me, although the vehemence came as a surprise. “Still, all the criticism and attacks on our findings don’t bother me because I’m from Green Bay Wisconsin, and I’ve been a Packers fan for thirty years, and I know what it’s like to hang in there from one losing season to the next.” She thought it would take five to ten years for their findings to be fully vindicated, and she couldn’t wait for that day. Her case now was stronger than ever, she said. The recent discovery of microorganisms far below the Columbia River, in Washington State, gave her a lot of corroborating evidence for nano-life on Mars. No one expected to find nanobacteria a mile or more below the surface of the Earth, and no one knows how they started growing. Like their ancient Martian cousins, they live in basalt. More important, they are almost as small as the Martian nanofossils. Critics of the meteorite team insisted that the presumed nanofossils in ALH 84001 were much smaller than any organisms found on Earth – too small to be considered micro-organisms. Since the Columbia River discovery, that objection lost much of its force.
I wondered what kind of energy source for life could be found in rock a mile or two underground, where there is no sunlight, no lightning, no real heat from the Earth’s core. Some scientists think the source could be as simple as water passing over the basalt, which might cause a chemical reaction. If this is the case, the answer to the Genesis Question becomes simpler all the time; it appears that the rock bottom (so it might be said) requirements for life are even more minimal than scientists believed only a few years earlier. All you need is water and an energy source for life to emerge. Water might be running through subsurface basalt everywhere; the same thing might have happened on other planets, or even on asteroids; it might be happening now. There might be more ways for life to emerge than we now imagine – enough to suggest that life really is an inevitable outcome of chemistry and an inevitable part of the universe, predestined, as it were, but so simple that we hardly acknowledge the phenomenon for what it is.
David McKay is tall, slender, silver-haired, professorial, imposing. As the leader of the meteorite team, he is suspicious of outsiders and chooses his words with care. His office, where we met, is capacious, even by the standards of the sprawling Johnson Space Center, and the walls are lined to the ceiling with plaques, awards, degrees, citations, and a child’s squiggly drawing of a small Martian meteorite beside a large man labeled, “Dad.”
“We are still getting new data,” he said, as he snacked on a small bag of pretzels, eying me warily. He wasn’t exactly thrilled that I’d appeared in his lair; he was sensitive to criticism and assumed I was about to add my voice to the chorus of those who angrily criticized his findings. He was about to dismiss me – or so it seemed – but he thought again, and decided to test his case with me. “We are very excited about the data from the meteorite called Nakhla that fell in Egypt in 1971,” he said. “The British Museum had a piece the size of a potato, covered with fusion crust, which protects it from contamination. The problem with the Allan Hills meteorite, ALH 84001, is that it may have been contaminated with carbon or terrestrial bacteria. A chunk of the Nakhla meteorite came in here, to our lab, and we had permission to break it up and pass it out to various investigators. We requested six grams. We think it’s likely to have the least contamination of any Martian meteorite.” I sensed he knew more, but this partial revelation was all he would risk revealing at the time.
He also wanted me to know he hadn’t given up on ALH 84001 as the prime suspect in the search for life on Mars. He didn’t want me to think for one second that Nakhla was a substitute for ALH 84001; rather, it offered supporting evidence. As he talked, it became apparent that he felt that all the criticisms leveled at his findings, and there had been a lot of them, more than most scientists encounter in a lifetime, had only strengthened the arguments he originally advanced. To illustrate what he meant, he invited me to sit with him before a large monitor. “Here’s a new picture from the Allan Hills meteorite. We really suspect these are fossilized bacteria. They have better characteristics than what we have already seen; they are curved, segmented. If you gave this to a biologist, he’d say, ‘Of course it’s bacteria,’ but we have to prove beyond a shadow of a doubt it’s of Martian origin and fossilized. Fossilization is very common with bacteria; the organic components are replaced by mineral components such as iron oxide or silica. This can happen quickly, in a couple of weeks, and it happens when you bury the material in water. They are one hundred to two hundred nanometers long and forty to fifty nanometers wide, smaller than the big worms in the published pictures, which were five hundred nanometers long. My guess is that life is still on Mars, but it’s underground, in the water system. That’s where the underground organisms are living, a couple of kilometers underground. On Earth,” he reminded me, “there are microbes growing four kilometers underground.”
As we parted, David McKay insisted, “Our critics have proved nothing. Our research has defeated each and every one of their arguments, and the case for ancient life on Mars is now stronger than ever.”
Nine months after our meeting, McKay made his latest findings public at the 1999 Lunar and Planetary Science Conference in Houston; his announcement added to the controversy and ensured that the debate surrounding fossilized Martian bacteria would continue for years. To his way of thinking, there were now two meteorites from Mars bearing evidence of fossilized bacteria, ALH 84001 and the newcomer, from Nakhla, Egypt. His detractors claimed his analysis of the newer meteorite, Nakhla, compounded the errors he had made in his analysis of the first, but his supporters insisted it offered compelling confirmation of extraterrestrial life.
3 GROUND TRUTH (#ulink_9dd2d5f6-be70-531c-a359-c187ff70c38d)
To reach the Jet Propulsion Laboratory, you take the freeway to Pasadena and get off at the Oak Grove Exit, then follow Oak Grove as it winds gently toward the mountains through the luxuriant landscape. You feel the smog settle on your chest as you go. There’s no suggestion of high technology in the area, just a somnolent Southern California suburb, lush, green, and slightly sullen. As you sense the end of the road approaching, you assess the looming mountains, but there’s still no sign of JPL, and you begin to wonder what gives. JPL isn’t exactly off-limits, but it’s not easily accessible, either. It will be found only by those who put some thought into looking for it. You think you’re finally there when several large white modern structures appear on the left, but as you drive up to them, you realize it’s a local high school, and then, just ahead, there’s a gate and a guardhouse, and that, at last, is JPL.
People arrive for work early. By 7:30 AM, the parking lot is filled with Hondas and Fords and Nissans and Tauruses – nothing fancy, except the odd Corvette. Employees quietly fan out across the campus and go to work. The buildings at JPL are boxy, functional, crisp. Within its offices, there are the same horrible green plants you see everywhere at NASA, at headquarters or the Johnson Space Center in Houston. Once you’re indoors, you can forget all about Southern California; you might as well be in Washington or Florida; it’s NASA-land.
Despite its innocuous location, JPL is among the world’s leading centers for spacecraft engineering and development. Started in 1936 as the Guggenheim Aeronautical Laboratory at the California Institute of Technology, JPL is now run jointly by NASA and Caltech. In the early days, there were just a few people on hand, including Frank Malina, a rocket enthusiast, and Theodore von Kármán, an influential Caltech professor. The lab barely survived the Depression, but it got a boost during World War II for experiments in rocketry. During the fifties, JPL developed a satellite that, according to legend, could have beaten Sputnik into orbit by a few months and irrevocably changed the space race – if it had been launched. Throughout the sixties, JPL solidified its reputation as the place for robotics – unmanned spacecraft destined for the moon and the planets – but it lacked the high profile of the Johnson Space Center in Houston or the Kennedy Space Center in Florida.
All that changed with the advent of the new Mars program in 1992, when a new generation of employees began streaming into JPL, reinvigorating the place. Unlike many of the old timers, they hadn’t come out of the military or the aerospace industry, they were just out of grad school, and had grown up watching the space program on television. They were young, and they weren’t burdened by the past. The men wore earrings and pony tails instead of military buzz cuts, and tie-dyed t-shirts replaced white polyester short-sleeve button-down shirts and narrow black ties. But that was just the men. Many of the new recruits were women, and among them was Jennifer Harris.
Growing up on her family’s farm in Fostoria, Ohio, Jennifer never expected to explore Mars or to become a flight manager for a Mars mission. She wanted to be a concert pianist. She played the piano, the saxophone, marimbas, bassoon, trumpet, tuba; she was a one-woman band. On the other hand, she loved math and competed successfully in county-wide math competitions. Astrophysics excited her imagination, especially black holes; she loved just thinking about them. In the summer before her senior year in high school, she went to music camp, where she realized that her survival as a concert pianist would depend on her ability to practice every waking moment, and she wasn’t sure that was what she wanted to do with her life. She also wanted to travel, to meet people; she was even thinking of becoming a missionary. When MIT accepted her, she went into a mild state of shock. Eventually, she chose to major in Aerospace Engineering – partly because it sounded like the coolest thing she could do and partly because her father had tested missiles for NASA when he was younger, and she had come of age hearing his tales of countdowns, halts, and explosions. Or maybe the picture of a rocket on a wall in the den of her home influenced her decision. After graduation, she went to work for the Jet Propulsion Laboratory.
Even after she arrived at JPL, Jennifer was restless. They were designing spacecraft on spec, hoping to get funding from Congress, and most projects never did. If a project actually received a green light, the lead time was awfully long. As she toiled away at her subsystems, she couldn’t see where her little cog fit into the machine, or if there even was a machine. She began to ask herself, “Is this all there is?”
She was single and didn’t have any serious ties to Pasadena or JPL. She chose to take a leave of absence, without assurance that a job would be waiting for her when she returned, if she returned. She still wanted to see the world and meet people, so she decided to do missionary work in Russia. She was assigned to Sevastapol, in the Crimea, near the Black Sea, where the conditions were unbelievably grim. There was no hot water, and they lived in cement buildings that were always cold and damp. A lot of the population were flat-out atheists. The economic situation was horrendous. She was paid about $30 a week, which made her among the wealthiest citizens of the town. Everyone around her was subsisting in a barter economy, using coupons instead of cash; one Snickers bar, for instance, cost 2,000 coupons. She and her friends based everything on the cost of a Snickers bar, but that didn’t help keep track of finances, because the inflation was incredible. Pretty soon that Snickers bar cost 8,000 coupons, then 16,000. People who had saved throughout their entire lives lost their fortunes overnight when the ruble crashed.
At times she wondered what kind of space program the Russians could possibly mount under these conditions. She had to wonder how they got anything done. As if the Russians’ pervasive fatalism wasn’t enough, there was the corruption, another thing she hadn’t been exposed to back at MIT and JPL and the family farm. She knew evil when she saw it, though, and it seemed to her that Russia, or at least her speck of it, was basically run by the Mafia, the politicians, and the church, all in bed together. After a while, she wondered if she was meant to be doing missionary work, if it was really the best use of her abilities. Was this what God wanted her to do? Was this what she wanted to do? She had to say honestly that the answer was no, her education was going to waste here. When her tour of duty was over, she left Russia to wander around Europe.
One day, she sent a postcard to a friend at JPL to say she would be back in a few months. “Do you have any jobs?” she asked, knowing the answer was very much in doubt. The day she arrived back in Ohio, JPL called to say they had a job for her, a good job, if she wanted it, but she would have to make a decision that day or the next. The job opening was on the new Pathfinder project, the next spacecraft to go to Mars. She said she’d take it. Jennifer was fairly skeptical about Pathfinder, but so was JPL. “A lot of people thought it would never work. There were so many things that could go wrong, especially with the Mars environment.” Her new job didn’t seem to have official status at JPL. Even the official Mars program people kept their distance. The development of Pathfinder struck her as a skunkworks, basically. She knew what that meant: if it wasn’t working, they could take it out and shoot it and bury it and no one would be the wiser.
The nature of her job changed as the mission went along. She began by working on software, “but the neat thing about Pathfinder was that once you took a job, it was sort of a ‘where-do-you-fit-in?’ type of thing. People didn’t say, ‘That’s not your job, stay out of there.’ They allowed you to move around, so I ended up doing more integration and testing in the early stages than operations. People were always given the opportunity to move over the borders and learn more and do more.” This open-ended, go-wherever-you-fit-in approach was something very new at NASA, and at JPL, which functioned along rigid, bureaucratic lines of command. The problem with the traditional structure was that if one element was delayed, or failed, or went awry, it brought the entire system to a halt. It became accepted practice for missions to slip several years. People were confined to narrowly defined jobs, and many of their talents and interests went untapped, because they had only a single task to perform. That paradigm didn’t apply to Pathfinder. Things were more flexible. It actually was faster and better and cheaper. This was all new, and very un-NASA.
Not everyone at JPL took to this open-ended approach, but Jennifer did. She became more confident in her various roles, accustomed to change. After her experiences in Russia, she knew not to overreact to situations and to plug along until she found a solution or failed miserably. In time she developed an informal network of specialists and advisors she could trust, her go-to people. The Pathfinder cradle-to-grave approach helped a lot. People came on board at the beginning, when the hardware was delivered, and they stayed all the way through to the end of operations. On the typical NASA mission, the person responsible for delivering the hardware would say, “I’ve delivered my hardware on time,” and walk away. If the hardware happened to be a camera, and it took pictures, they felt they had achieved their goal. They didn’t care if it was impossible to operate, or if it didn’t get the right pictures. But if you worked on Pathfinder, you had to undergo a mental shift. If you designed your component incorrectly, if it was difficult to test or to operate, it was still your problem.
It was difficult to explain the new thinking, Jennifer realized. You had to experience it for yourself, and then it could make a huge impact. You would become committed to the ultimate goal, whatever it was. In Pathfinder, the goal was to get to Mars quickly and cheaply, and to get a rover to function on the Martian terrain. Things worked in a sort of non-systematic way because people attacked problems where they saw them. Eventually, they generated procedures, and she wrote the documentation, but this was not a document-heavy mission, like most NASA missions. She sat down with a couple of other people, and they asked, “What are the most likely contingencies? What’s our nominal plan at the big-picture level?” She realized this could be a wonderful opportunity to participate in the exploration of space, and that idea pleased her greatly. “I feel like God has blessed me in my career,” she once wrote, “and I would like to glorify Him by exploring His incredible creation.” So the missionary had a new mission, but even as a scientist, especially as a scientist, she still devoted herself to God.
The Pathfinder mission originated in a speech given by President George Bush in 1989 to commemorate the twentieth anniversary of men – American men! – landing on the moon. NASA was in the doldrums at the time; and the occasion of the speech seemed to point up how little it had done since the halcyon days of Apollo. The Challenger disaster, which occurred more than three years before the anniversary, still loomed; when people thought of NASA, they didn’t visualize Neil Armstrong jumping onto the surface of the moon, they thought of the faces of the parents of Christa McAuliffe, the school teacher who rode aboard the Space Shuttle, looking in disbelief at the Y trail left in the sky by the catastrophic explosion.
Along came George Bush, discussing the future of space exploration. The demoralized NASA contingent could scarcely believe what they heard. Did the President mention “the permanent settlement of space”? Yes, he did. Did he also say it was time to travel “back to the moon, back to the future, and this time back to stay”? Indeed, he said that, as well. But surely he could not have said, “And then, a journey into tomorrow, a journey to another planet: a manned mission to Mars.” Yes! The President said that, too. Mars. The NASA bureaucrats began to ask themselves: how much was all this going to cost? No one thought you could go back to the moon and on to Mars for under 400 billion dollars; the tasks might require twice that amount. NASA’s annual budget at the time was around 13 billion. Where would the money come from? Interestingly, few doubted that the technology existed to send people to Mars, or that it could be developed quickly; if NASA had the money, they could get the job done.
George Bush’s remarks evoked John Kennedy’s famous speech in which he charged NASA with the duty of sending men to the moon. Without realizing it, Bush tapped into the agency’s other obsession, reaching Mars, an obsession that had begun in the mind of its ace rocket engineer, Wernher von Braun, during World War II. Von Braun, a member of the Nazi party, and a favorite of Hitler’s, had helped to design the V-2 missile. When he became disillusioned with the Nazi war machine, the Gestapo arrested him and sent him to jail. In his cell, he turned his attention to interplanetary travel, and Mars in particular. And it was in these strange and harsh circumstances that the kernel of what would become the American effort to explore Mars was born. In May 1945, von Braun and over a hundred other German rocket scientists surrendered to the Allies. They were swiftly transplanted to New Mexico to continue their work on rockets, this time for the United States. The German V-2 became the prototype of a new generation of American missiles, and on the strength of his engineering accomplishments for the Nazis, von Braun quickly established himself as the chief architect of the American space program’s booster rockets during the 1950s and 1960s; his designs were responsible for getting American men to the moon.
Throughout his career, von Braun was mesmerized by Mars. He published his plan to send people to Mars, the one he had conceived in jail, as a long magazine article titled “Das Marsprojekt,” which was translated into English. In 1953, it appeared as a book in the United States: The Mars Project. It became a classic, but this was not science fiction; The Mars Project contained no inspiring rhetoric about humankind’s greatest adventure. It was a how-to manual, a master plan for getting people to Mars. He used a simple slide rule to make his calculations, and its pages contained his blueprint for the actual mission, using available technology. “The logistic requirements for a large elaborate expedition to Mars are no greater than for those for a minor military operation extending over a limited theater of war,” he wrote. The key to reaching Mars, he believed, was sending a flotilla of spacecraft. “I believe it is time to explode once and for all the theory of the solitary space rocket and its little band of bold interplanetary travelers. No such lonesome, extra-orbital thermos bottle will ever escape Earth’s gravity and drift toward Mars.” Instead, in von Braun’s vision, “Each ship of the flotilla will be assembled in a two-hour orbital path around the earth, to which three-stage ferry rockets will deliver all the necessary components. Once the vessels are assembled, fueled, and ‘in all respects ready for space,’ they will leave this ‘orbit of departure’ and begin a voyage which will take them out of the earth’s field of gravity and set them into an elliptical orbit around the sun … Three of the vessels will be equipped with ‘landing boats’ for descent to Mars’s surface. Of these three boats, two will return to the circum-Martian orbit after shedding the wings which enabled them to use the Martian atmosphere for a glider landing. The landing party will be trans-shipped to the seven interplanetary vessels, together with the crews of the three which bore the landing boats and whatever Martian materials have been gathered. The two boats and the three ships which bore them will be abandoned in the circum-Martian orbit, and the entire personnel will return to Earth orbit in the seven remaining planetary ships. From this orbit, the men will return to the earth’s surface by the upper stages of the same three-stage ferry vessels which served to build and equip the space ships.” It was a grand scheme, and it became the template for NASA’s plans to send people to Mars, a goal von Braun thought could be accomplished by the late 1970s.
Bush’s speech endowed von Braun’s dormant plan with new life, but the prospect of returning to Mars raised new questions, as well. If NASA planned to send people to Mars safely, scientists needed to know much more about the Red Planet. If there was life on Mars, what form did it take? Was it dangerous to humans? Could it devastate the Earth if astronauts brought samples home? How severe were the effects of radiation? And, most important, was there water on Mars? The presence of water would dramatically enhance the prospects for finding life, but more than that, water meant it would be possible to manufacture rocket fuel, oxygen, and other human essentials on Mars.
Three years after Bush’s speech, in 1992, NASA announced plans to send between twelve and twenty small landers to Mars. They would fly frequently, and they would take advantage of new equipment, especially computer technology, to explore more effectively. The new program went by the name of Mars Environmental Survey – MESUR, in NASA-speak. The agency then announced another planetary program, Discovery, with similar goals; it was a nice instance of the right hand not knowing what the left was doing. Eventually, the two programs merged into one trial program: Pathfinder. It was going to be fast, it was going to be cheap, but no one knew if it would be better than previous planetary missions. Unlike most NASA missions, which are built and often operated by a private aerospace contractor such as Lockheed Martin, Pathfinder was an in-house project, designed, built, and operated by JPL. It was meant to embody JPL’s prowess as NASA’s robotics center, and that posed an embarrassing problem.
It had been a generation since Americans had landed a spacecraft on Mars. The old guard was gone, and few around JPL or NASA remembered exactly how that trick worked. Some scientific data had been preserved, though not completely, along with thousands of Viking images, but there was little documentation of the mission’s engineering accomplishments. Rob Manning, the young leader of Pathfinder’s Entry, Descent, and Landing team, sought veterans who could tell him what they had done on Viking, but many had died, and others had retired. JPL pulled a few of the old grizzlies out of retirement to help assemble a unit capable of developing a lander, and they went to work under Manning.
The idea behind Pathfinder, to develop and build a new spacecraft on a drastically reduced schedule and budget to land on the surface of Mars, sounded like a losing proposition to many at JPL, given the risks involved in getting there. Just setting their ship safely onto the surface posed difficult engineering problems. The spacecraft travels at about 17,000 miles an hour as it reaches Mars. Then it must slow to nearly zero miles an hour so that it does not vaporize in the Martian atmosphere or crash into the surface like a meteorite. The Viking solution to this problem, an expensive and cumbersome one, employed powerful, heavy thrusters capable of guiding the spacecraft gently to the surface. There was no money for that kind of extravagance with Pathfinder. Instead, Pathfinder’s engineers planned to wrap the lander in a protective bubble, place the bubble inside an aerodynamic cone, and parachute it through Mars’ thin atmosphere to the surface, letting the cone peel off in sections. Then Pathfinder would bounce around the surface like a big hi-tech beach ball. If all these cushioning devices worked properly, Pathfinder would still be in once piece when it came to a stop. This follow-the-bouncing spacecraft approach was profoundly troubling to conservative NASA engineers, but Manning casually accepted the risks. “Pathfinder is just a rotating bullet with nothing controlling it. This cone shape produces some unstable results – not so unstable that it’s devastating, but you live with that.” When he presented his landing scheme to NASA’s review board, they were, he said, “skeptical – borderline hostile, as they should be. They were paid to challenge everything. So it was a big deal when we deviated from the Viking heritage.”
Even if it landed safely, Pathfinder wouldn’t sit still on the surface of Mars, taking measurements, as the Viking landers had. It would carry a rover designed to roam across the surface, functioning as a twelve-inch-tall geologist. This was not a new idea; for decades, NASA had explored the possibility of sending a rover to investigate Mars. “My most persistent emotion in working with the Viking lander pictures was frustration at our immobility,” Carl Sagan recalled in 1980. “I found myself unconsciously urging the spacecraft at least to stand on its tiptoes, as if this laboratory, designed for immobility, were perversely refusing to manage even a little hop. How we longed to poke that dune with the sample arm, look for life beneath the rock, see if that distant ridge was a crater rampart … I know a hundred places on Mars which are far more interesting than our landing sites. The ideal tool is a roving vehicle carrying on advanced experiments, particularly in imaging, chemistry and biology.” He outlined, with his usual visionary fervor, a rover-based mission very much like Pathfinder. “It is within our capability to land a rover on Mars that could scan its surroundings, see the most interesting place in its field of view and, by the same time tomorrow, be there … Public interest in such a mission would be sizable. Every day a set of new vistas would arrive on our home television screens. We could trace the route, ponder the findings, suggest new destinations … A billion people could participate in the exploration of another world.” At the time he wrote those words, they sounded like the vaguest hyperbole, but Pathfinder and the Internet would make his outlandish prediction a reality.
Although a rover seemed like a nifty idea, it was untried. The later flights in the Apollo program had taken along a dune buggy to traverse the powdery surface of the moon. The astronauts could steer and stop the rickety lunar flivver at will. The difficulties involved in guiding Pathfinder’s rover across the surface of Mars by remote control seemed insurmountable. What if the rover didn’t emerge from the beach ball after all that bouncing? What if it got stuck on a rock or a crevice or sank into the talcum-powder-fine Martian soil? What if the beach ball landed in inhospitable terrain? What if it landed on the wrong part of Mars, where it couldn’t receive signals from Earth? And yet, if it avoided all these pitfalls and worked, the rover would provide a whole new paradigm for exploring the surface of Mars, because JPL had visions of building bigger and better rovers in years to come, until they reached the size of small trucks. But most people guessed a small rover would never work, not with the two million dollars allotted for its development.
A debate sprang up over the best way to control the rover, and, given the personalities involved, it quickly escalated into a dispute over technological theology. Tony Spear, a veteran engineer at JPL, believed the most reliable and cheapest way was to tether it to the mother ship. The other approach, advocated by Donna Shirley, was to control the rover remotely, but that meant designing or finding a new radio system, one that could tolerate the extreme fluctuations in the Martian environment, including fluctuations in temperature between the rover and the lander.
Donna Shirley was a controversial figure around JPL. When her name was announced as the Pathfinder mission director, a few cheers went up, but only a few; there was also consternation. Tony Spear, the Pathfinder project manager, was nowhere to be seen during the announcement, and Donna took his absence to indicate his lack of support. She could live with that. She thought the apparent indifference had to do with the fact that she was a woman, but she was accustomed to handling that problem. Donna had been with JPL since 1966, when very few women filled responsible posts there; during her years there, she married, raised a daughter, and got a divorce. At work, she was relentlessly cheerful, almost, but not quite, to the point of bullying, and she was a world-class talker. Many bureaucrats and scientists at NASA were camera shy, but when a television crew appeared at JPL, there was Donna Shirley in her bright red dress, flashing her assertive smile, prepared to discuss in her folksy Oklahoma twang just about anything. Her appearance was perfect for television. TV producers were delighted to interview the ebullient Donna Shirley instead of a pale male attired in the gray suit, gold-rimmed glasses, and neat mustache favored by the upper echelons at NASA. But, while being interviewed, she occasionally appeared to take credit for the work of a great many NASA scientists and engineers toiling anonymously, and that did not work to her advantage.
Her detractors said she really didn’t know her science well, but she made her lack of expertise into an asset because she had no scientific agenda, nothing to prove. She was content to bang heads together cheerfully and say, “Look, guys, now we are going to do it this way.” To the increasing number of women coming out of graduate school to work for NASA, she became a symbol. These younger women liked to tell a story about the time Donna Shirley attended a launch party at Cape Canaveral. As usual in those days, she was the only woman present. A guitarist singing a bawdy song, accompanying himself on the guitar, stopped dead when he saw her. She took his guitar and completed the song herself, delighting everyone. That was great, as far things went, but she didn’t realize there was a tradition at these launch parties that a woman – a hooker, basically – was paid to show up and pull a stunt like that. One of the men assumed Donna had been hired for the occasion, maneuvered her into an alcove, and grabbed her. “I didn’t exactly deck him,” she said, “I just hit him on the nose.”
Working on Pathfinder, she saw her team of engineers and scientists as a large family, her family. To her credit, she encouraged everyone to talk to everyone else, if only in self-defense, and she always smiled and radiated optimism. Most found it impossible to bear a grudge for long in the face of such cheerfulness; it was too exhausting to oppose her. Still, she wanted her radio-controlled rover for Pathfinder, and Tony Spear, the project manager, did not. “In his position, I wouldn’t either,” she said, “because he had the impossible job of landing on Mars for a fraction of what it cost the last time we landed. He had no idea how to do it, and here’s this parasite coming along, giving him nothing but trouble. What I did was to convince the scientists that we really could do useful work with the rover. That was number one. Number two was to convince Tony that we really could fly without damaging his mission.” When Donna presented her case to NASA’s review board, one member, Jim Martin, the former Viking project manager, insisted a Mars landing could not cost less than Viking had. As for the rover, “he thought it was terrible.” Donna and the rover team persisted, building better iterations of the rover and demonstrating they worked as advertised. “It became a very powerful selling tool,” she realized, and eventually, to everyone’s surprise, it turned into the mission’s raison d’être.
If Pathfinder’s engineering was, ultimately, carefully weighed, the mission’s science component tended to be rushed, improvised, an afterthought. Plenty of scientists were eager to participate in the new Mars mission, but they needed time and money to formulate, conduct, and analyze experiments. Pathfinder didn’t work that way. At the last minute, for instance NASA stuck a couple of stereographic cameras on the lander and another camera on the rover. These weren’t your standard television cameras; they used a technology known as a Charge Couple Device. The CCD reproduces light very accurately and is especially useful for spectroscopy, which reveals more than the naked eye can see by measuring which wavelengths of light are absorbed, and which reflected, from an object. They were useful, but they were not capable of sending back the sparkling, gorgeous images returned by Viking twenty years earlier. Pathfinder also carried an Alpha Proton X-ray spectrometer to detect the composition of Martian rocks, and a weather mast to measure the Martian temperature and atmospheric conditions. Every so often, Pathfinder would collect the weather mast’s data and return it to Earth, so for the first time it would be possible to obtain accurate weather reports from the surface of Mars. Everyone agreed the weather mast would be a terrific experiment, if it worked. It looked like Pathfinder had a chance to become a real mission, after all.
Manning’s team conducted early Pathfinder landing tests at a NASA facility in Cleveland, Ohio, which featured a large vacuum chamber. Within, girders, lava rocks, and wood simulated the Martian surface. They dropped Pathfinder in its protective bubble onto the sharp objects and observed the result.
R-r-r-r-r-r-rip!
“The first time we did it, we had a tear the size of a human being,” Manning said. They took it back to the lab, fixed it up, and dropped it again.
R-r-r-r-r-r-rip!
They tweaked it and tried again. R-r-r-r-r-r-rip! … R-r-r-r-r-r-rip! … R-r-r-r-r-r-rip!
The trials went on like that for months; they were “total disasters,” said Manning, and NASA nearly canceled the mission. Late in 1995, the Pathfinder team redoubled its efforts. The engineers adjusted the spacecraft’s small guidance rockets. They modified the shape of the sphere contained inside the protective beach ball. They had been imitating the Russian model, which was spherical and consequently difficult to manufacture; now they adopted a tetrahedron, which was easier to manufacture. They toyed with the air bags protecting the tetrahedron, trying one deflation strategy after another, getting incremental improvements. Gradually, they came to feel more confident about Pathfinder. They did have one advantage: because the gravity of Mars is less than half of Earth’s, the spacecraft would endure less wear and tear. “We always worked in terms of the mass, and the mass kept getting bigger and bigger,” Donna said. “That meant the mechanical parts had to be heavier because they were supporting all of this additional structure. The mission design people came to the rescue. They said, ‘Okay, if we’re going to fly into the atmosphere of Mars, there’s a corridor we have to hit. If we go in too shallow, we’ll just skip out of the atmosphere and keep on going. If we go in too deep, we’ll burn up on entry, or we won’t have enough atmosphere to slow down before we hit the surface.’ So there’s a narrow range of angles at which you can enter the atmosphere, and that takes some really accurate shooting by the navigators. So the navigators heard this and said, ‘Okay, if we can shoot more accurately and give up some of our margin for error, we can let the spacecraft have more mass.’” Now the engineers were able to add small thrusters that would slow Pathfinder during its descent to the surface.
The mission was still alive, but the development of a decent, affordable rover still posed engineering problems. JPL had to devise a nimble mechanical creature that could scale small barriers and climb over rocks, like a little tank. To complicate matters, it would take twelve or fifteen minutes for a radio signal to travel from the Earth to Mars, which eliminated spontaneous, real time commands. “If you’re looking through the rover’s eyes, and you see a cliff coming, and you say, ‘Stop!’ it’s too late – it will be over the cliff, so it has to be smart enough to stay out of trouble,” Shirley said. In addition to negotiating the Martian terrain, which was in many details unknown, the rover had to keep its solar panels in position to receive sunlight, or it would lose power and die.
Attempting to meet these requirements, JPL devised variations on a theme. They built a rover the size of a small truck, and they built one just eight inches long, nicknamed “Tooth.” They built a mid-sized rover called Rocky, which, when tested in the desert, actually did things required on Mars, such as scooping up soil. Rocky went through various iterations until it weighed just fifteen pounds, yet negotiated the kind of obstacles and terrain that geologists expected to find on the surface of Mars. It could perform simple experiments, and it appeared sturdy enough to withstand the rigors of landing on the Martian surface and bouncing around inside a beach ball.
The development of Pathfinder’s components took place in a knowledge vacuum, because the engineers and scientists didn’t know exactly where they were going on Mars or what to expect when they got there. From a spacecraft’s point of view, Mars presents a landscape of treachery. The team expected to receive finely detailed studies of the surface from Mars Observer, the billion-dollar spacecraft launched on September 25, 1992. It was supposed to reach Mars the following August, when its cameras would send back pictures of the Martian surface with much higher resolution than Viking had captured in the seventies, and those pictures were supposed to give JPL a well-informed notion of where to land their bouncing beach ball. Just when Mars Observer was to begin orbiting around the Red Planet, JPL lost the signal, and the spacecraft was never heard from again. There was speculation that a fuel line had frozen and ruptured, and the spacecraft went out of control, but nobody could say for sure – nobody, that is, but fringe elements, who concocted some fairly creative theories. There was the “Hey! That was no accident” scenario: NASA deliberately destroyed the spacecraft because it had detected signs of intelligent life on Mars. And there was the “Mad Martian” scenario: Mars Observer had been destroyed by sophisticated Martian weapons whose existence NASA conspired to conceal from the American public.
Within NASA, scientists feared they had lost their chance to return to Mars. Shortly after Mars Observer disappeared, Dan Goldin journeyed to the Goddard Space Flight Center in Greenbelt, Maryland, to rally the troops. Although Goddard is only a short commute from NASA headquarters in Washington, D.C., the head of NASA is not in the habit of dropping in, so his presence signaled a major announcement. For many scientists, it was their first close-up look at the man whom George Bush had appointed in 1992 to run the agency. At Goddard, he reminded the scientists that NASA attempts to do difficult things, risky things, and the possibility of losing a spacecraft was an ever-present hazard, but the risk didn’t mean the mission wasn’t worth doing. They would continue to explore Mars. Conditioned to regard managers as antagonists, the scientists were impressed.
Under Goldin’s leadership, the loss of Mars Observer provoked NASA to hone and intensify its Martian agenda. The agency decided to launch a pair of missions to the Red Planet approximately every two years, whenever the orbits of the two planets brought them into a favorable alignment, beginning in 1998. Each mission would have a distinct identity and purpose, but, taken as a whole, they would culminate in sending humans to the Red Planet. What sounded like a rather vague statement of intent acquired sudden conviction in August 1996, with the announcement of possible fossilized life in ALH 84001. Goldin suddenly began pressing JPL and the scientists to make specific plans to bring a sample of Martian soil back to Earth to continue the search for life. Donna Shirley and the other managers said they couldn’t do that much on their subsistence budget.
Returning a sample of Mars to Earth is a complex, costly, and hazardous undertaking. You send two spacecraft – a lander and orbiter – to Mars. The lander scoops up enough soil to fill a can of Coke, and then it must launch itself from the surface of the Red Planet and guide itself to a rendezvous with the orbiter. NASA has never done that before – launched a spacecraft from a distant planet. If that part of the mission succeeded, the orbiter would bring the sample to Earth, where new hazards would arise – for instance, the sample might be dangerous or even lethal to terrestrial life. The safe handling, testing, and decontamination of the sample would amount to a large project in itself. NASA confronted a similar problem with samples of the moon in the sixties, and set up an elaborate, isolated lunar laboratory at the Johnson Space Center, where moon rocks were analyzed with great care by technicians wearing long rubber sleeves and working behind glass until the rocks were found to be harmless. There is much greater concern about possible harmful effects of Martian soil because of the greater likelihood of life on Mars. The quarantine will likely be extreme and long-lasting. When you talk about a sample return, you’re talking about spending billions of dollars and placing the lives of everyone on the planet in some degree of jeopardy. You’re talking about a mission almost as complicated as a human mission to Mars.
NASA expanded its string of Mars missions into a more formal, and better-funded, program of exploration. “The Human Exploration people at the Johnson Space Center came along and said, ‘Okay, we want to fly humans to Mars.’ Dan Goldin set 2018 as a date, but the Johnson Space Center said, ‘Well, we think it should be earlier than that. We’d like to do it by 2011,’” Donna Shirley said. “To decide whether to send humans to Mars by 2011, you need to make a decision by about 2005 that you are going to invest in doing that, and you need to have the information necessary to make the decision. The only way to get the answers by 2005 is to fly by 2001.” Just when it looked as though Mars might get a lot more money, Congress realized that the International Space Station was generating huge cost overruns, and it sucked up money that might have gone to human Mars exploration.
Goldin didn’t give up on the idea of sending people to Mars. He directed scientists at NASA to make plans for an eventual human mission. Although the project was unfunded and unofficial, it was real enough, and the scientists and engineers went at it with the zeal of true believers. Their enterprise went under vague names, such as Beyond Earth Orbit (BEO) and Human Exploration and Development of Space (HEDS), names that meant different things to different people, and wouldn’t upset Congress. But to those within NASA, the names meant one thing: sending people to Mars. So a lot was riding on the success of the little Pathfinder mission; the implications went far beyond the success or failure of its experiments. It was, potentially, the first step in the most ambitious exploration in history, but few outside of NASA realized that.
The loss of Mars Observer meant Pathfinder’s site selection team was forced to rely on twenty-year-old Viking data. Since Pathfinder was designed to plummet to the Martian surface, it would not be able pick and choose a landing site as Viking had. The site would have to be selected in advance, and it had better be good. A lot of the responsibility for selecting a site fell to Matt Golombek, a young geologist. If you can recall the kid in the seventh grade who always seemed a couple of steps ahead of the teacher, let alone the class, and who was wiry and agile and had a way of laughing off anything that bothered him, you have a sense of Matt Golombek. He came to the agency from Rutgers and the University of Massachusetts as one of the new generation of planetary geologists that included Maria Zuber and Jim Garvin. “You only do this because you love it. It’s not like you’re going to get rich or famous. You’re especially not going to get rich,” he says. Although he reports to work at JPL, which is a government facility, he is, like everyone else there, technically employed by Caltech. It’s a peculiar arrangement, which he facetiously likens to a “money-laundering scheme to lower the number of civil servants.” Matt maintains a certain skepticism concerning government work. “You know what they say about civil servants, don’t you? They’re like rusty old guns. They don’t work, and you can’t fire them.”
Despite his youth, Matt brought with him long experience in Mars exploration. “I was the pre-Project Scientist on all the Mars missions before Pathfinder for ten years, and there was a whole string of them. I was brought in originally with something called the Mars Rover Sample Return, which was actually a politically motivated study to work with the Russians, which didn’t go anywhere.” This was followed by assignments on other luckless missions, including Mars Observer. “I think one of the reasons they assigned me to Pathfinder as the Project Scientist was that I was young. Part of their thinking was, ‘Well, it doesn’t matter who we appoint. It’s not going to mean anything.’ I wasn’t sure I even wanted the job, because the mission was an entry, descent, and landing demonstration that would have little or no science of benefit to anyone. What the hell do you need a Project Scientist for? There’s no science, right? I mean, Pathfinder’s main goal was to land safely, period.”
To achieve even that limited goal, he spent two years mastering every detail of the choices before making his recommendation. The pixels in the old Viking images concealed many potential hazards. “Imagine if you looked at an image to select a potential landing site, and the smallest you see is the size of a football stadium, and you are worried about things that are the size of a meter,” Matt said. “All we had was very coarse, low resolution remote sensing information about Mars, yet we had to guesstimate that the place we would come to rest would be safe, and that the rover could travel out on it. That’s a very difficult job. It was a two-and-a-half year process. We did an exhaustive study of the options, of cost, and of the kind of science you could get at different places.” He had to factor many subtle requirements into his choice. He looked for a spot where Pathfinder’s solar cells would supply power, and where the antennae could communicate with Earth as often as possible. He wanted an area free of mesas, which would confuse Pathfinder’s navigational system. Those and other constraints eliminated ninety percent of the surface of the planet. Geological factors eliminated a number of other tempting targets; if an area was too dusty, too cavernous, or too rocky, it was eliminated from consideration.
There was something else on his mind. What was the point in going all the way to Mars only to land in a dried-up, featureless lake bed and watch the rover go round and round? Why not use the tools they were bringing, the Alpha Proton X-ray spectrometer and the cameras? Why not make Pathfinder a science mission as well as an engineering mission? “Wait a minute,” he told anyone who’d listen, “we can actually do science.” Perhaps the mission would need a Project Scientist, after all. Matt saw his chance to push against the system and work with the engineers to make room for science. For Pathfinder to accomplish anything significant, it would have to land in a place with attention-grabbing rocks – rocks that would speak volumes about Martian geological history, especially the presence of water, rocks that were big, but not too big. He didn’t want boulders, for instance, and he didn’t want pebbles, either. He wanted, so he said, a “rock mission.” He wanted a “grab bag, a smorgasbord, a potpourri of rocks.” He wanted sermons in stone.
Everyone at JPL recognized that Matt was a very good scientist. Now he demonstrated that he was a very good scientific operator, as well. His gift for caustic repartee concealed considerable shrewdness; depending on his purposes, he could be engagingly cynical, or firm and cool. He was persuasive with his colleagues, lacing his remarks with irony, imparting to all those around him the intoxicating sense that they were being drawn into some grand cosmic joke. Nothing intimidated him, least of all NASA’s bureaucracy. NASA was a bunch of civil servants – c’mon, people, don’t you see the joke in this situation? It was a racket. Caltech was another racket, as was JPL. Then there was the science racket, the engineering racket, the budget racket, and of course, the Mars racket, and they were all susceptible to lobbying and influence if you knew where to apply pressure, which came down to motivating people to do something different. “The hardest part of going to Mars,” Matt once told me, “was getting everyone working on Pathfinder to march in the same direction.”
Unlike most scientists, he was good with the engineers; he appreciated the difficulties they faced. Scientists and engineers often develop adversarial relationships: scientists usually display scant patience for the difficulties of building and operating the instruments, and engineers tend to regard scientists as impractical, arrogant, impossible to please. Stepping into the midst of the fray, Matt pushed back on the scientists, knocking down the number of experiments, and he convinced engineers they could do things they wouldn’t have thought possible. That was a formula for a very successful manager of space science. “You almost have to turn yourself into an engineer,” he said, “because you have to understand what your spacecraft’s doing. Your dominant job as a Project Scientist is to make sure they don’t engineer the science off the mission. It’s not that engineers are dumb, they’re doing the best they can, but they don’t necessarily think about science. And so you sit through interminable meetings waiting for the one silly thing that will pop up and threaten the science. I mean, it’s crazy! The other aspect, once you get the mission going, is that you have to lead the science team. You have to show them where you’re going. What’s really important? How do you allocate resources? How do you keep people’s egos from getting in the way? That’s very tricky.”
He became adept at building a consensus around the selection of a site. He led a site selection workshop at the Johnson Space Center in Houston, fielding ideas from the entire Mars community. They whittled the choices down to about ten, which Matt put on a large, complicated diagram called “The Chart from Hell.” After much study, Matt, working with another geologist, Hank Moore, concentrated on a Martian basin named Chryse Planitia – Chryse Plain. Within Chryse there is an outflow channel called Ares Vallis, the geological legacy of a huge, ancient flood that deposited interesting and varied rocks on the surface. The diverse rocks were the greatest attraction, as far as he was concerned. The area’s sheer size made it very appealing. Pathfinder, in addition to all its other uncertainties, could not make a carefully predetermined landing; if all went well, it would land somewhere within an ellipse 60 miles wide and twice as long. Matt fretted over the temperature range of Ares Vallis, over the distribution of rocks, and especially over the amount of dust blowing around. If you’ve ever come into contact with terrestrial lava dust, you immediately understand the problem. It’s gritty and irritating and clings to the fingers. Martian dust, made from powdered lava, is similarly fine and gritty. It relentlessly clogs machinery and obscures solar panels.
To get a better idea of what Pathfinder might encounter if it landed in Ares Vallis, Matt used an Earth analogue – not Iceland, in this case, but the Channeled Scabland in the state of Washington. This desolate region was formed during a huge flood about 13,000 years ago; the turbulent water redeposited rocks across a flat plain, just as Matt believed had once occurred in Ares Vallis on Mars. The Channeled Scabland is much smaller than the Martian site he was considering, and tufts of grass spring from the soil, but geologically, it is remarkably similar to Ares Vallis. He took several field trips to the Channeled Scabland, and even brought along the rover to see how it would fare on the rock-strewn terrain. It ably negotiated the varied surface, and he figured he had finally found his landing site. Ares Vallis was safe, it was geologically interesting, and it was, he hoped, not too dusty.
NASA’s review panel considered his choice. “You’re going to go back there and kill the spacecraft – and kill your career,” they said, but Matt would not be intimidated. He had seen the results of Pathfinder tests in even worse conditions than it would encounter on Mars, and the spacecraft had survived. “We ended up making the most robust lander that’s ever been designed to land on a planet. Pathfinder could land anywhere,” he told the panel. In the end, Matt got his way, and his landing site on Ares Vallis, but his career would ride on Pathfinder’s fortunes.
4 FROM OUTER SPACE TO CYBERSPACE (#ulink_e41eb43a-6b46-502c-a96f-58888afa1c0c)
The Kennedy Space Center in Florida employs sixteen thousand people and covers over a hundred thousand acres. It includes a wildlife refuge; herons, egrets, condors, crocodiles, horses, and cattle roam its expanses. From the road, you can see a six-foot-wide bald eagle’s nest suspended in the branches of a tree. If you drive in the general direction of the launch pads, away from the animals, you will see the outsized Vehicle Assembly Building, a giant hangar for rocket ships, shimmering through the haze. You can pick out the odd Shuttle transporter here and there; they resemble huge, primitive locomotives with giant cleats. Many of the transporters are rusting in the humidity. In recent years, NASA has built the center into a tourist attraction featuring life-size mockups of the Space Shuttle, a few garish exhibits, and a souvenir emporium. On the outskirts of Titusville, the nearest town, discarded rockets litter front yards like so many abandoned cars, looking nothing like the towers of power that I remember from my youth. A heavy nostalgia for the future lingers over the place like the scent of magnolia on a humid evening, and the marquee in front of the local high school always reads, “Countdown to Graduation – Six Weeks.”
This was where Pathfinder, weighing nearly a ton, arrived on a rainy day in August of 1996. There was a lot of work to do. Things were just beginning to get serious at this point. First thing, engineers wiped down the spacecraft with alcohol to prevent bacteria from Earth contaminating the surface of Mars. Then, electrical technicians wearing bunny suits to prevent dust or hair falling into the delicate machinery, took the spacecraft into a large clean room and tested every circuit. They corrected software problems and installed the rover’s heaters, which contained a tiny amount of plutonium. NASA wasn’t eager to advertise the fact, for the use of nuclear materials in space, even for purely scientific purposes, rouses environmentalists to fury. It was also a giant bureaucratic pain, because NASA had to prepare exhaustive environmental impact statements. The plutonium was deemed necessary because of Mars’ great distance from the Sun. On Mars, sunlight is only a quarter of the strength that it is on Earth, and small solar cells alone could not generate enough power to operate even a small spacecraft and rover.
After the initial preparation, Pathfinder underwent months of additional testing at Kennedy. Often, the tests were more complicated than the actual mission would be. For a test to work correctly, the ground team had to simulate the positioning of the stars and the Sun, the amount of light, and the temperature for Pathfinder, and then program Pathfinder to respond. Nothing went exactly as planned; everything required extra effort. Work became so intense that the young engineers involved with the project didn’t know what to do when they weren’t testing Pathfinder; they sought any distraction available in greater Titusville. They screamed themselves hoarse in a Karaoke bar, they played in mud volleyball tournaments, they surfed, they picnicked in the rain – anything to take their minds off the obstacles they faced to ready Pathfinder for space. At four o’clock one morning, they attended a Shuttle launch. The rocket’s glare turned night into day, and the sound of its engines was powerful enough to make observers’ clothes tremble. Pathfinder’s launch vehicle, a Delta II built by Boeing, was nowhere near as big as the Shuttle’s powerful solid state boosters, but the Shuttle simply attains low Earth orbit, about 350 miles high. It circles the Earth for a few days, and then lands on a nice smooth airstrip. If you happen to be a planetary exploration zealot, Shuttle missions, for all their sound and fury, can be a trifle dull. Pathfinder, in contrast, would travel 309 million miles to reach Mars, following the broad ellipse of its trajectory, and would arrive in a new world.
Whenever they could find a few minutes to spare, Pathfinder’s youthful team members posted their field journals on the Internet. These were casual, subjective reflections on their lives and work, with more questions than answers. The mere act of writing made everyone self-aware. It was weird: they were doing their jobs, and simultaneously, they were watching themselves do their jobs. They were doing their jobs on Pathfinder, and they were watching themselves at the same time. Anyone with access to the Internet could log on and check up on the team members’ psyches. As with so much else on this mission, the plan was very cool, and very un-NASA. Taken together, the team’s written observations sounded like an all-night college dormitory bull session about the meaning of God and life and truth and beauty, and that was their charm. The team members, especially the younger ones, unashamedly asked, Who am I? Where am I going? The field journals became confessional, a form of therapy. The more enthusiastic correspondents realized something unusual was going on here, something that extended beyond the boundaries of the Pathfinder mission, something that the words “faster, better, cheaper” didn’t begin to convey: a transformation of consciousness. They weren’t just devising a new way to reach Mars, although that was surely foremost in their minds. They were collaborating on a new way to solve problems, to create, to communicate, to imagine.
In late October, the engineers at KSC mated the spacecraft with the cruise stage of the Delta rocket, and loaded fuel into the spacecraft’s propulsion system. It was powered by hydrazine, nasty stuff that requires careful handling. If you touch hydrazine, it can burn your skin. If you spill it, it can start a fire. The engineers wore protective suits very much like an astronaut’s while they worked. In this instance, the fueling process proceeded safely. Soon after, technicians tested the Deep Space Network, the system for communicating with Pathfinder. The DSN consists of three ground stations – one in Goldstone, California, another in Madrid, Spain, and a third in Canberra, Australia. Exquisitely sensitive, the DSN can pick up exceptionally faint signals from spacecraft as far away as Jupiter, and possibly beyond the boundaries of the Solar System. In November, the team started holding practice countdowns. The launch was approaching rapidly; now it was days, not weeks away. Back at JPL in Pasadena, a sign read, “OBJECTS ON THE CALENDAR ARE CLOSER THAN THEY APPEAR.” It was a message that everyone on the Pathfinder team had learned to heed.
The closing of the spacecraft, supposedly a modest episode in the life of Pathfinder, inflated into a media event. Dan Goldin, the NASA Administrator, showed up at the Kennedy Space Center and gave a rousing speech. Crowds turned out to watch Pathfinder’s four petals fold shut around the rover. As everyone applauded, the engineers glimpsed a sliver of daylight between the petals. This was not a good omen: to close a spacecraft in preparation for launch, only to find that the pieces don’t fit. The event had been scheduled for television broadcast, but the engineers waved away the cameras so they could study the problem. This was the first time the spacecraft had been fully loaded, and the increased weight created structural sagging, which kept the petals from sealing as designed. During the next several days, engineers worked desperately to repair the problem, and couriers bearing modified parts flew in from JPL. “objects on the calendar are closer than they appear.” One by one the new parts were mated, and the entire assembly was stacked on to the launch vehicle, and trucked over to Launch Pad 17A at Cape Canaveral Air Station.
The launch was scheduled for December 2, and it proved to be an exercise in frustration. “The weather was so bad they decided to cancel the attempt for the day,” Donna Shirley wrote in her field journal. “On December 3, many of us went out to the launch pad to watch the gantry roll back. This was supposed to happen at 5 PM but didn’t actually occur until 7:30 PM. It got colder and colder, and there was a prelaunch party scheduled. A few diehards, including me, were all that were left to see the rocket standing free of the supporting structure. It was worth waiting for, shining in the spotlights, gleaming blue and white.” For a few hours that night, the countdown proceeded smoothly, but problems started to mount. “First, the winds aloft looked bad,” Donna recorded. “The range sent up balloon after balloon to see what the winds were like, and gradually they began to improve. By the fourth balloon they looked acceptable, and we all began to get excited. But there was another problem. One of the ground computers was having problems. After much discussion, the launch vehicle team decided to change to a backup computer. But about two minutes before launch time, that computer also had trouble, and the launch was scrubbed. Everyone sagged. We’d been running on adrenalin, not a bit sleepy, but once there was no launch everyone went home to bed.” Bridget Landry, a Pathfinder software engineer who’d been following the tortured countdown from Pasadena, also turned to her journal for consolation: “We were all so disappointed when they said we had to scrub! All that anticipation! In some ways, it’s funny, all that buildup, and then nothing happens. But it’s also scary: the Russian mission, Mars ’96, was unable to escape Earth’s gravity just a few weeks ago. Somehow, that makes us worry more about our launch. Guess scientists and engineers can be a little superstitious, just like anyone else.”
On December 4, with all conditions favorable, Pathfinder finally launched from Cape Canaveral at 1:58 AM, local time. Although the blast was visible in the night sky for miles, there were no throngs along the beach. The event was the merest blip on the news radar; the public remained mostly oblivious to the fact that we were returning to Mars. Pathfinder was just another planetary mission, for the time being.
After launch, the engineers at JPL had a rough idea where Pathfinder was located in space, but now they needed to know precisely where it was. To get a better fix on the spacecraft, they planned to establish contact with it through the Deep Space Network. But before they could do that, the spacecraft was supposed to orient itself with a device called a sun sensor, a small disc covered with photoelectric cells. The event was supposed to occur about ninety minutes after the launch, with the spacecraft traveling at 17,000 miles an hour, but the sensor wasn’t working properly, and if it failed, they would soon lose the spacecraft entirely. As everyone involved with the mission knew, if Pathfinder failed, the future of the entire Mars program, including a human mission, would be in grave doubt.
After extensive searches, the Goldstone, California, antenna of the Deep Space Network finally acquired a signal from Pathfinder, which confirmed that something was seriously wrong with the spacecraft: the sun sensor wasn’t returning data. The best guess was that the sensor’s photoelectric cells had been nearly blinded by exhaust from one of the launch rockets, and Pathfinder refused to pay attention to it. The fix was simple, in theory: send three new software files to command Pathfinder to tune into the sensor. The engineers transmitted the files again and again, but each time they were only partially received, and no one could say why. The situation, already very serious, deteriorated when the spacecraft began to ignore all commands. Eventually, one of the engineers realized that Pathfinder was revolving slowly and came in position to receive commands for only five seconds at a time. Now Pathfinder had two problems – the dirty sensor and the uncontrollable spinning. The double fault was likely to be fatal to the entire mission.
Working around the clock, the Pathfinder engineers compensated for the rotation, and transmitted a complete set of commands to the spacecraft. Once they did, Pathfinder paid attention to the sun sensor, oriented itself properly, and both problems disappeared. The process of resolving them, collaborative and critical, proved to be a rite of passage for the Pathfinder team. Although they were relieved to have fixed a problem that could have killed the mission, they knew that more could appear at any time. Pathfinder was single-string all the way; if a component failed, there was no backup.
In the ensuing days, a weird sense of calm descended on JPL. There wasn’t much anyone could do for the next ten months besides monitor the spacecraft, whose instruments were powered down for the long cruise. “I’ve always known that the spirit on Pathfinder was special,” Bridget Landry noted in her journal, “but when the people who worked on Apollo 11 and 13 say this project has more sense of identity and team spirit than even those two missions, you know you’re involved with something extraordinary. But the feeling here, at least for me, is bittersweet, too. Now that we’ve launched, some people are being laid off, and even though most of us are staying, the scope of the mission means that in less than a year, all this will be over.”
In the spring of 1997, as the spacecraft approached Mars, the Pathfinder team began a new series of tests to prepare for its prime mission – the weeks Pathfinder would spend on the Red Planet, roving and returning data. “Think of it as a rehearsal,” Bridget Landry’s journal explained. “We have a computer that simulates the spacecraft, as well as a model of the lander and a duplicate rover. We put the last two in our sandbox (a room full of sand and rocks used to simulate the surface of Mars), then close the curtains so that no one can see in, and a few people go in and rearrange the rocks. Then the operations team has to take pictures with the lander camera, determine where the rocks are, and generally do all the tasks we’ll do on the first two days on Mars.”
They tried to maintain a sense of humor; any joke was better than no joke. The technician who ran the sandbox – the gremlin, they called him – planted toy Martians amid the simulated rocks, and the controllers logged the little creatures as they would in an actual mission. The tests increased in frequency until they took place nearly all the time, and Bridget, for one, felt overwhelmed by the intensity. “I refer to this as ‘a snake swallowing a gopher’: it is an enormous amount of data/work/whatever, and it can be tracked visibly as the lump makes its way through the system.”
By this point, the team members had left terrestrial time behind; they lived on Martian time. A Martian day, or sol, is forty minutes longer than an Earth day; every twenty-four hours, the team migrated forty minutes around the clock to remain in synchronization with the Red Planet. The time shift was very disorienting; after a while, they began to feel as though they were living on Mars. “I had no clue as to what time it was on Earth, although I could tell you what sol it was,” Bridget recorded. “I even tried to date a check with the sol number during this time, and I dated leftover food in the refrigerator with the sol. I totally lost track of time. I couldn’t tell you what planet I was on, never mind what day it was. I was often surprised to walk out of JPL and find the sun up.” While she worked the graveyard shift, she found it “strange to be driving home under rosy skies, pulling the pillow over my head to shut out the morning light. Stranger still to hear meetings called for midnight or 2 AM, and having to ask whether an event scheduled for 6 means AM or PM.” One night, while driving home on the freeway, Bridget fell asleep at the wheel. There was no accident, no one was hurt, fortunately, but the mishap terrified her. After that, she kept a sleeping bag and pillow in her office so she could sleep there, if she needed.
Bridget’s role on Pathfinder changed along with her schedule, and throughout the project, she never knew what to expect. Her first job was straightforward: adapting sequencing software to Pathfinder’s commands and instruments, but then she worked with the imaging team in a job so idiosyncratic there wasn’t even a name for it. For official purposes, she called herself an Uplink System Engineer, but the title didn’t begin to describe the nuances of the position. After a while, she came to see the benefits of this elastic, improvisational approach; it was liberating, as long as she could keep up with it. “I think this is the main reason we succeeded – there was so little redundancy in staff and hardware that you had to understand not only your little piece of the puzzle, but how it all fit together. There was no one else to do it. If people couldn’t think this way, they left.” She managed to hang on, but found her new job staggeringly intricate and pressured. If just one system on which she worked malfunctioned, the entire mission could go haywire. “I always mean to go back and clean up the code I write, but there is only so much time and money and energy – you do the things that matter most, until it’s good enough. Striving for perfection is a good and worthwhile effort. Expecting actually to attain perfection can kill you.”
What started out as a cool new job – but still, just a job – became much more than that to Bridget and to the other team members. Most were young, this was their first big mission, in some cases their first job at NASA, and while they were confident of their technical skills, which was the reason they were hired in the first place, they were less certain they could manage the emotional strain. That was something they didn’t teach you back at Caltech or MIT: how to deal with failure and uncertainty. “This is a nerve-wracking experience,” Rob Manning confided in his journal. “It is really tough to go to another planet.” Normally a jovial soul, Rob endured nightmares. In one, “I launched my dog Scooter up in the spacecraft, and it landed on Mars. I realized, too late, how was I going to get him home? I was really upset for letting him go!”
On Monday, June 23, David Mittman, the new Pathfinder flight controller, awoke at three o’clock in the morning and showered in the dark so as not to disturb his sleeping family. He had managed a few minutes extra sleep by shaving the night before. He dressed, and drove to work at JPL. The place was already alive; June 23 was going to be a crucial day in the life of Pathfinder, which would land on Mars on July 4. “All the flight engineers have been developing ‘sequences’ – collections of commands executed in order by the spacecraft – in preparation for landing,” he wrote. “These sequences have gone through many reviews and revisions. Some sequences have been changed as many as twenty times as we’ve learned more about how to operate Mars Pathfinder.” Mittman and his team were about to send nearly 300 sequences to Pathfinder via the Deep Space Network’s transmitter in Madrid. The task was time consuming, because each sequence, traveling at the speed of light, as fast as anything can go in the universe, required nine minutes and forty seconds to reach Pathfinder as it sped toward Mars. The transmission proceeded smoothly for nearly twelve hours, until glitches in terrestrial equipment halted the proceedings.
Конец ознакомительного фрагмента.
Текст предоставлен ООО «ЛитРес».
Прочитайте эту книгу целиком, купив полную легальную версию (https://www.litres.ru/laurence-bergreen/the-quest-for-mars-nasa-scientists-and-their-search-for/) на ЛитРес.
Безопасно оплатить книгу можно банковской картой Visa, MasterCard, Maestro, со счета мобильного телефона, с платежного терминала, в салоне МТС или Связной, через PayPal, WebMoney, Яндекс.Деньги, QIWI Кошелек, бонусными картами или другим удобным Вам способом.
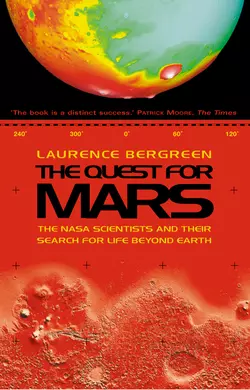
The Quest for Mars: NASA scientists and Their Search for Life Beyond Earth
Laurence Bergreen
Тип: электронная книга
Жанр: Зарубежная образовательная литература
Язык: на английском языке
Издательство: HarperCollins
Дата публикации: 16.04.2024
Отзывы: Пока нет Добавить отзыв
О книге: This edition does not include illustrations.Is there life on Mars? And if not, why not? These questions have gripped mankind throughout the twentieth century. In the shadow of the new millennium, The Quest for Mars seeks the definitive answers from the dedicated NASA scientists participating in the race to discover life on the Red Planet.‘Ever since I was a small child, I’ve believed there was life out there. When I look at the magnitude of the universe, with its billions of stars, I believe that if life developed here on Earth, it must have developed elsewhere. We simply can’t be unique. I really don’t think we’re the most intelligent life forms in the universe, but that’s just my gut feeling’, Dr Claire Parkinson, NASA scientist.Of all the planets, Mars has exerted the most powerful allure over the human intellect and imagination. Generations of astronomers have expected to find clues to the origin and destiny of Earth and its inhabitants concealed amid the red storms sweeping across the surface of Mars. Today, the mystique of Mars is even greater. Public interest in the Mars mission is sky-high; the exploits of the tiny Mars Rover ‘Sojourner’ in the summer of 1997 excited the greatest curiosity in a space mission in a generation.In The Quest for Mars Laurence Bergreen has unrestricted access to a team of NASA employees – engineers, geologists and other scientists – who are consumed by the search for proof of life on Mars. As one formidable obstacle after another attempts to scupper their quest for a deeper understanding of life on Mars and throughout the Solar System, the narrative takes us step by step through the exhilaration and the despondency of their extraordinary adventure. Nothing is off limits in this unique, behind-the-scenes story of space exploration.